Introduction
- Introduction
- Geological Background
- Magma Formation
- Triggering Mechanisms
- Types of Volcanic Eruptions
- Effusive Eruptions
- Explosive Eruptions
- Phreatic Eruptions
- The Role of Volcanic Vent Structure
- Fissure Vents
- Monitoring Volcanic Activity
- Seismographs
- Gas Emission Measurements
- Ground Deformation Monitoring
- Preparing for Volcanic Eruptions
- Case Studies
- Environmental Impacts
- Socio-economic Effects
- Conclusion
- FAQs
Volcanoes, geological wonders that punctuate the Earth’s surface, stand as both marvels of nature and potential sources of destruction. Defining these natural phenomena and comprehending the mechanisms behind their eruptions hold paramount importance for humanity’s safety and scientific understanding.
Geological Background
Understanding the geological context in which volcanoes arise provides crucial insights into their behavior and impact. The Earth’s structure is composed of various layers, with the crust being the outermost layer. Beneath the crust lies the mantle, a semi-fluid layer of rock. The crust is divided into several large pieces called tectonic plates, which float on the semi-fluid mantle and move slowly over time.
The movement of these tectonic plates plays a pivotal role in the formation and activity of volcanoes. When two plates converge, one may be forced beneath the other in a process known as subduction. Alternatively, when plates diverge, magma from the mantle can rise to the surface, creating new crust. Additionally, plates sliding past each other can generate intense friction and heat, leading to volcanic activity along fault lines.
One of the key factors in volcanic eruptions is the formation of magma chambers beneath the Earth’s surface. Magma, a molten mixture of rock, minerals, and gases, forms deep within the Earth’s mantle. As tectonic plates shift and interact, magma can be pushed upward through cracks and fissures in the crust, accumulating in underground chambers known as magma chambers. These chambers serve as reservoirs for molten rock, providing the potential fuel for volcanic eruptions when pressure builds and magma finds a path to the surface.
Magma Formation
Magma, the molten rock that fuels volcanic eruptions, forms through a complex process influenced by various factors. Understanding the composition of magma and the factors that influence its formation is essential for predicting volcanic activity and assessing its potential hazards.
Composition of Magma
Magma is composed of three primary components: liquid rock, solid mineral crystals, and dissolved gases. The exact composition of magma varies depending on factors such as the type of rock involved, the temperature and pressure conditions, and the presence of volatile elements.
The liquid portion of magma consists mainly of silicate minerals, such as quartz, feldspar, and mica, which melt at high temperatures. These minerals give magma its characteristic viscosity, or resistance to flow, which can range from relatively low for basaltic magma to extremely high for rhyolitic magma.
In addition to the liquid portion, magma often contains solid mineral crystals that form as the magma cools and solidifies. These crystals can range in size from microscopic to several centimeters in diameter and are typically composed of the same minerals found in the liquid portion of the magma.
Dissolved gases, such as water vapor, carbon dioxide, and sulfur dioxide, are also present in magma. These gases can exert pressure on the surrounding rock and contribute to the explosive nature of volcanic eruptions.
Factors Influencing Magma Formation
Several factors influence the formation of magma, including temperature, pressure, and the composition of the rocks involved.
Temperature plays a critical role in magma formation, as it determines the melting point of the rocks involved. In general, rocks melt at higher temperatures deeper within the Earth’s mantle and crust, where temperatures are hotter. As rocks are subjected to increasing temperatures due to processes such as mantle convection or tectonic activity, they may begin to melt and form magma.
Pressure also influences magma formation, as increasing pressure tends to raise the melting point of rocks. This is why magma tends to form at greater depths within the Earth, where pressures are higher. However, in some cases, a decrease in pressure can trigger the melting of rocks, such as when tectonic forces cause rocks to rise towards the Earth’s surface.
The composition of the rocks involved is another crucial factor in magma formation. Different types of rocks have different melting points and behaviors when subjected to heat and pressure. For example, mafic rocks such as basalt tend to melt at lower temperatures than felsic rocks such as granite. As a result, the composition of the rocks in a given area can determine the type of magma that forms and the style of volcanic eruptions that occur.
Overall, the formation of magma is a complex process influenced by a combination of temperature, pressure, and rock composition. By studying these factors, scientists can gain valuable insights into the behavior of volcanoes and improve our ability to predict and mitigate the hazards they pose.
Triggering Mechanisms
Volcanic eruptions are often triggered by various mechanisms that disturb the delicate balance within the Earth’s crust and mantle. Understanding these triggering mechanisms is crucial for predicting volcanic activity and mitigating its potential impacts on human populations and the environment.
Pressure Buildup
One of the primary triggering mechanisms for volcanic eruptions is the buildup of pressure within magma chambers beneath the Earth’s surface. As magma accumulates in these underground reservoirs, it exerts increasing pressure on the surrounding rock. Eventually, this pressure can reach a critical point where it exceeds the strength of the overlying rock, leading to fractures and the release of volcanic gases.
The release of pressure can trigger the sudden expansion of gases dissolved in the magma, causing it to rise rapidly towards the surface. This process, known as decompression melting, can lead to explosive volcanic eruptions characterized by the violent expulsion of ash, rock fragments, and gases.
Water Infiltration
Water infiltration into volcanic vents can also act as a triggering mechanism for eruptions, particularly in areas with abundant groundwater or nearby bodies of water. When water comes into contact with hot volcanic rocks, it can flash into steam, causing a rapid increase in pressure within the volcanic system.
The sudden expansion of steam can fracture the surrounding rock and create pathways for magma to ascend towards the surface. This process, known as phreatic or steam-driven eruption, can produce powerful explosions of steam, ash, and volcanic gases without any warning signs.
Tectonic Activity
Tectonic activity along fault lines and plate boundaries is another common triggering mechanism for volcanic eruptions. When tectonic plates interact, they can generate intense friction and heat, leading to the melting of rocks and the formation of magma beneath the Earth’s surface.
Subduction zones, where one tectonic plate is forced beneath another, are particularly prone to volcanic activity. The subduction of oceanic crust beneath continental crust can lead to the formation of magma chambers and the subsequent eruption of volcanoes along the overriding plate.
Additionally, the rifting of tectonic plates can create new volcanic vents as magma rises to fill the void created by the separation of the plates. This process, known as rift volcanism, is responsible for the formation of volcanic features such as mid-ocean ridges and rift valleys.
In conclusion, volcanic eruptions are complex phenomena driven by a combination of factors, including pressure buildup within magma chambers, water infiltration into volcanic vents, and tectonic activity along fault lines and plate boundaries. By studying these triggering mechanisms, scientists can improve our understanding of volcanic processes and enhance our ability to forecast and mitigate the hazards posed by volcanic eruptions.
Types of Volcanic Eruptions
Volcanic eruptions come in various forms, each characterized by its own distinct features and hazards. Understanding the different types of volcanic eruptions is essential for assessing their potential impacts on surrounding communities and environments.
Effusive Eruptions
Effusive eruptions are characterized by the relatively gentle outpouring of lava from volcanic vents. Unlike explosive eruptions, which involve the violent release of gases and fragmented rock, effusive eruptions produce lava flows that slowly advance across the landscape.
Effusive eruptions typically occur at shield volcanoes, which have broad, gently sloping profiles due to the low viscosity of their lava. The lava emitted during these eruptions is often basaltic in composition, meaning it is rich in iron and magnesium and flows easily when molten.
While effusive eruptions are generally less hazardous to human life and property compared to explosive eruptions, they can still pose risks to nearby communities, particularly if lava flows threaten populated areas or infrastructure.
Explosive Eruptions
Explosive eruptions are the most violent type of volcanic activity, characterized by the sudden release of pressure from highly viscous magma trapped beneath the Earth’s surface. These eruptions produce dramatic explosions of ash, rock fragments, and volcanic gases, which can reach heights of tens of kilometers into the atmosphere.
Explosive eruptions often occur at stratovolcanoes, also known as composite volcanoes, which are steep-sided cones built up by alternating layers of lava, ash, and other volcanic debris. The magma involved in these eruptions is typically rich in silica, which increases its viscosity and promotes explosive behavior.
The hazards associated with explosive eruptions include pyroclastic flows, fast-moving avalanches of hot gas and volcanic debris that can travel at speeds of hundreds of kilometers per hour, as well as ash fall, which can disrupt air travel and cause respiratory problems for nearby populations.
Phreatic Eruptions
Phreatic eruptions, also known as steam-driven eruptions, occur when groundwater comes into contact with hot volcanic rocks, causing it to flash into steam. These eruptions can produce sudden explosions of steam, ash, and volcanic gases without any warning signs, making them particularly hazardous to nearby communities.
Phreatic eruptions often occur at volcanic vents that have been sealed by solidified lava or other volcanic debris, preventing magma from reaching the surface. When groundwater seeps into these vents and comes into contact with hot rocks, it rapidly turns into steam, causing pressure to build up until it is released in a sudden explosion.
While phreatic eruptions are generally less destructive than effusive or explosive eruptions, they can still pose risks to nearby populations, particularly if they occur without warning. Additionally, the ash and gases emitted during these eruptions can have environmental and health impacts on surrounding ecosystems and communities.
In conclusion, the three main types of volcanic eruptions – effusive, explosive, and phreatic – each have their own distinct characteristics and hazards. By understanding these different types of eruptions, scientists can better assess the potential risks posed by volcanic activity and develop strategies to mitigate their impacts on human populations and the environment.
Effusive Eruptions
Effusive eruptions are characterized by the relatively gentle outpouring of lava from volcanic vents. Unlike explosive eruptions, which involve the violent release of gases and fragmented rock, effusive eruptions produce lava flows that slowly advance across the landscape.
Characteristics
- Low Viscosity: The lava involved in effusive eruptions is typically low in silica content, which results in low viscosity. This low viscosity allows the lava to flow easily and spread out over large areas, creating extensive lava fields.
- Steady Flow: Effusive eruptions are often characterized by a steady, continuous flow of lava from the volcanic vent. Unlike explosive eruptions, which can be short-lived and highly unpredictable, effusive eruptions can last for weeks, months, or even years.
- Shield-shaped Profiles: The lava emitted during effusive eruptions tends to accumulate around the vent in a broad, gently sloping mound known as a shield volcano. These volcanoes have a characteristic shield-shaped profile, with shallow slopes that extend for tens or even hundreds of kilometers from the central vent.
- Lava Tubes: Effusive eruptions can sometimes form lava tubes, which are underground channels through which lava flows beneath the surface. These lava tubes can transport lava over long distances, allowing it to travel downslope and spread out over flat terrain.
- Minimal Explosive Activity: Unlike explosive eruptions, which generate significant amounts of volcanic ash and gases, effusive eruptions typically produce minimal explosive activity. Instead, the lava flows steadily from the vent, gradually building up the shield volcano’s size and shape.
Examples: Shield Volcanoes
Some of the most famous examples of shield volcanoes around the world include:
- Mauna Loa: Located on the island of Hawaii, Mauna Loa is one of the largest shield volcanoes on Earth. It rises over 13,000 feet above sea level and covers an area of more than 2,000 square miles. Mauna Loa has been the site of numerous effusive eruptions throughout history, producing vast lava flows that have added to the island’s landmass.
- Mauna Kea: Also located on the island of Hawaii, Mauna Kea is another massive shield volcano that rises over 13,000 feet above sea level. Although Mauna Kea is currently dormant, it has experienced numerous effusive eruptions in the past, contributing to the island’s diverse volcanic landscape.
- Galápagos Islands: The Galápagos Islands, located in the Pacific Ocean off the coast of Ecuador, are home to several shield volcanoes, including Fernandina, Sierra Negra, and Alcedo. These volcanoes have been the site of numerous effusive eruptions, shaping the unique ecosystems of the Galápagos Islands and providing valuable insights into the process of volcanic island formation.
In conclusion, effusive eruptions are characterized by the steady flow of lava from volcanic vents, resulting in the formation of shield volcanoes with broad, gently sloping profiles. Understanding the characteristics and examples of effusive eruptions is essential for comprehending the diverse range of volcanic activity observed around the world.
Explosive Eruptions
Explosive eruptions are among the most dramatic and hazardous volcanic events, characterized by the sudden release of pressure from highly viscous magma trapped beneath the Earth’s surface. These eruptions often result in violent explosions of ash, rock fragments, and volcanic gases, posing significant risks to nearby communities and the environment.
Characteristics
- High Viscosity: The magma involved in explosive eruptions is typically rich in silica, which increases its viscosity or resistance to flow. This high viscosity prevents gases from escaping easily, leading to the buildup of pressure within the magma chamber.
- Violent Explosions: Explosive eruptions are marked by sudden, violent explosions as the pressure within the magma chamber exceeds the strength of the overlying rock. These explosions can eject vast quantities of ash, rock fragments, and volcanic gases into the atmosphere at high velocities, creating volcanic plumes that can reach heights of tens of kilometers.
- Pyroclastic Flows: One of the most hazardous aspects of explosive eruptions is the generation of pyroclastic flows, fast-moving avalanches of hot gas and volcanic debris that can travel at speeds of hundreds of kilometers per hour. These deadly flows can devastate everything in their path, including buildings, forests, and infrastructure.
- Ash Fall: Explosive eruptions can also produce widespread ash fall, coating the landscape with a layer of fine volcanic ash that can disrupt air travel, contaminate water sources, and cause respiratory problems for nearby populations. The weight of accumulated ash can also collapse roofs and damage crops, leading to significant economic losses.
- Secondary Hazards: In addition to the primary hazards associated with explosive eruptions, such as pyroclastic flows and ash fall, these events can also trigger secondary hazards such as lahars (volcanic mudflows), landslides, and volcanic lightning. These secondary hazards can further exacerbate the impacts of the eruption and pose additional risks to human life and property.
Examples: Stratovolcanoes
Some of the most famous examples of stratovolcanoes around the world include:
- Mount Vesuvius: Located near the city of Naples in Italy, Mount Vesuvius is one of the most well-known and dangerous stratovolcanoes in the world. It famously erupted in 79 AD, burying the ancient cities of Pompeii and Herculaneum under a thick layer of ash and volcanic debris.
- Mount St. Helens: Located in the state of Washington in the United States, Mount St. Helens is another notable stratovolcano known for its explosive eruptions. It famously erupted in 1980, producing a massive eruption column that reached a height of over 24 kilometers and causing widespread devastation in the surrounding area.
- Mount Fuji: Located on the island of Honshu in Japan, Mount Fuji is an iconic stratovolcano and the highest peak in Japan. While it is currently dormant, Mount Fuji has experienced numerous explosive eruptions throughout history, shaping the landscape of central Japan and influencing the country’s culture and traditions.
In conclusion, explosive eruptions are characterized by violent explosions of ash, rock fragments, and volcanic gases, posing significant risks to nearby communities and the environment. Understanding the characteristics and examples of explosive eruptions is essential for assessing the potential hazards posed by volcanic activity and developing strategies to mitigate its impacts.
Phreatic Eruptions
Phreatic eruptions, also known as steam-driven eruptions, occur when groundwater comes into contact with hot volcanic rocks, causing it to flash into steam. These eruptions can produce sudden explosions of steam, ash, and volcanic gases without warning signs, posing significant hazards to nearby communities and the environment.
Characteristics
- Sudden Onset: Phreatic eruptions often occur suddenly and without warning. They are triggered when water infiltrates volcanic vents or comes into contact with hot volcanic rocks, causing it to rapidly turn into steam. This rapid conversion of water to steam generates intense pressure within the volcanic system, leading to explosive eruptions.
- Steam-driven Explosions: Phreatic eruptions are driven primarily by the expansion of steam as it rapidly expands within the volcanic vent. This expansion of steam fractures the surrounding rock and creates pathways for the release of volcanic gases and ash. The resulting explosion can propel volcanic debris and gases high into the atmosphere.
- Limited Magma Involvement: Unlike effusive or explosive eruptions, which involve the ascent and eruption of molten magma, phreatic eruptions typically involve little to no magma. Instead, these eruptions are driven by the interaction of groundwater with hot volcanic rocks, producing steam and other volcanic gases.
- Ash and Steam Plumes: Phreatic eruptions can produce dense plumes of steam, ash, and volcanic gases that rise rapidly into the atmosphere. These plumes can reach heights of several kilometers and spread over large areas, posing risks to aviation, air quality, and human health.
- Secondary Hazards: In addition to the primary hazards associated with phreatic eruptions, such as ash fall and steam-driven explosions, these events can also trigger secondary hazards such as lahars (volcanic mudflows), landslides, and debris flows. These secondary hazards can further exacerbate the impacts of the eruption and pose additional risks to nearby communities and infrastructure.
Examples: Steam-driven Eruptions
Some notable examples of steam-driven eruptions include:
- Whakaari / White Island: Located off the coast of New Zealand’s North Island, Whakaari / White Island is an active stratovolcano known for its frequent phreatic eruptions. In December 2019, a phreatic eruption occurred without warning, resulting in multiple fatalities and injuries among tourists visiting the island.
- Dieng Volcanic Complex: Situated in Central Java, Indonesia, the Dieng Volcanic Complex is known for its numerous volcanic vents and geothermal activity. Phreatic eruptions have occurred at various vents within the complex, producing steam and ash plumes that pose risks to nearby communities and agriculture.
- Yellowstone National Park: While not exclusively driven by phreatic activity, Yellowstone National Park in the United States is home to numerous hydrothermal features, including geysers, hot springs, and fumaroles. These features are fueled by the heat of volcanic activity beneath the surface and can occasionally experience phreatic eruptions.
In conclusion, phreatic eruptions are characterized by sudden explosions of steam, ash, and volcanic gases driven by the interaction of groundwater with hot volcanic rocks. Understanding the characteristics and examples of phreatic eruptions is essential for assessing the potential hazards posed by volcanic activity and implementing measures to mitigate their impacts on nearby communities and ecosystems.
The Role of Volcanic Vent Structure
The structure of volcanic vents plays a crucial role in determining the style and intensity of volcanic eruptions. Different types of vents, such as central vents and fissure vents, can influence how magma is expelled from the Earth’s interior and how volcanic material is distributed on the surface.
Central Vent
A central vent is a single opening at the summit or central part of a volcano through which magma and volcanic gases are expelled during eruptions. This type of vent is commonly associated with stratovolcanoes and shield volcanoes, as well as other types of volcanic edifices.
Characteristics of Central Vents:
- Focused Eruption: Central vents channel the ascent of magma from the Earth’s mantle to the surface through a single opening, resulting in a focused eruption. This focused eruption can produce lava flows, ash columns, and pyroclastic flows that emanate directly from the central vent.
- Formation of Crater: Over time, repeated eruptions from a central vent can lead to the formation of a crater or caldera at the summit of the volcano. This crater may contain a lava lake, a secondary vent, or other volcanic features resulting from past eruptions.
- Regulation of Eruption Style: The size and shape of the central vent can influence the style and intensity of volcanic eruptions. Narrow vents may produce explosive eruptions, while wider vents may allow for effusive eruptions characterized by the gentle outpouring of lava.
Fissure Vents
Fissure vents, also known as eruptive fissures or volcanic fissures, are long cracks or fractures in the Earth’s surface from which lava and volcanic gases are expelled during eruptions. These vents can extend for several kilometers and may be associated with both shield volcanoes and volcanic rift zones.
Characteristics of Fissure Vents:
- Linear Eruption: Fissure vents produce linear eruptions along the length of the fracture, resulting in the formation of elongated lava flows known as lava channels or lava tubes. These lava flows can extend for many kilometers from the fissure vent, covering vast areas of the surrounding landscape.
- Multiple Vent Locations: Fissure vents may consist of a single continuous fracture or multiple interconnected fractures. This allows for the simultaneous eruption of magma from multiple vent locations, increasing the overall volume of erupted material and the extent of the volcanic activity.
- Formation of Lava Plateaus: Eruptions from fissure vents can result in the formation of lava plateaus or flood basalts, extensive flat or gently sloping areas covered by thick layers of solidified lava. These lava plateaus are commonly associated with volcanic rift zones and can cover thousands of square kilometers.
In conclusion, the structure of volcanic vents, including central vents and fissure vents, plays a critical role in shaping the style and intensity of volcanic eruptions. Understanding the characteristics of these vents is essential for predicting volcanic activity and assessing the potential hazards posed by volcanic eruptions to nearby communities and infrastructure.
Monitoring Volcanic Activity
Monitoring volcanic activity is essential for predicting eruptions, assessing potential hazards, and mitigating risks to human populations and infrastructure. Scientists employ various techniques and instruments to monitor volcanoes, including seismographs, gas emission measurements, and ground deformation monitoring.
Seismographs
Seismographs are instruments used to detect and record ground motion caused by volcanic activity, such as earthquakes and tremors. These instruments consist of a seismometer, which detects ground motion, and a recording device, which records the seismic signals.
Role of Seismographs in Monitoring Volcanic Activity:
- Detection of Volcanic Tremors: Seismographs can detect small tremors and earthquakes associated with the movement of magma beneath the Earth’s surface. These tremors may indicate the movement of magma towards the surface and the potential for an impending eruption.
- Monitoring Volcanic Unrest: Seismographs provide real-time data on seismic activity, allowing scientists to monitor volcanic unrest and assess the likelihood of future eruptions. Changes in the frequency, intensity, and depth of seismic events can provide valuable insights into the dynamics of volcanic systems.
- Early Warning Systems: Seismographs play a crucial role in early warning systems for volcanic eruptions. By detecting precursory seismic signals, scientists can issue timely warnings to at-risk communities and implement evacuation measures to minimize the impact of an eruption.
Gas Emission Measurements
Gas emission measurements involve monitoring the composition and quantity of gases emitted by volcanoes, such as sulfur dioxide, carbon dioxide, and water vapor. These measurements provide valuable information about the activity and behavior of volcanic systems.
Role of Gas Emission Measurements in Monitoring Volcanic Activity:
- Detection of Volcanic Gas Plumes: Gas emission measurements can detect and quantify volcanic gas plumes emitted by active vents and fumaroles. Changes in the composition and quantity of gases can indicate variations in volcanic activity, such as the rise of magma towards the surface.
- Monitoring Degassing Processes: Gas emission measurements provide insights into the degassing processes occurring within volcanic systems, such as the release of gases from magma as it ascends through the Earth’s crust. Monitoring changes in gas composition and emission rates can help scientists understand the evolution of volcanic activity over time.
- Assessment of Hazards: Certain volcanic gases, such as sulfur dioxide, can pose significant health hazards to nearby communities and ecosystems. Gas emission measurements allow scientists to assess the potential risks posed by volcanic emissions and implement measures to protect public health and safety.
Ground Deformation Monitoring
Ground deformation monitoring involves measuring changes in the shape, elevation, and movement of the Earth’s surface caused by volcanic activity. These measurements are typically obtained using satellite-based remote sensing techniques, GPS receivers, and ground-based instruments.
Role of Ground Deformation Monitoring in Monitoring Volcanic Activity:
- Detection of Ground Swelling: Ground deformation monitoring can detect and quantify inflation or swelling of the Earth’s surface caused by the intrusion of magma beneath the volcano. These changes in ground elevation may precede volcanic eruptions and serve as precursory signals of increased volcanic activity.
- Assessment of Volcanic Hazards: Ground deformation measurements provide valuable information about the potential hazards posed by volcanic activity, such as the likelihood of eruptions, landslides, and lahars. By monitoring changes in ground elevation and movement, scientists can assess the risks to nearby communities and infrastructure.
- Prediction of Eruptions: Ground deformation monitoring plays a crucial role in predicting volcanic eruptions and issuing timely warnings to at-risk populations. By analyzing patterns of ground deformation over time, scientists can make informed predictions about the timing, location, and intensity of future eruptions.
In conclusion, monitoring volcanic activity using techniques such as seismographs, gas emission measurements, and ground deformation monitoring is essential for understanding the behavior of volcanic systems and mitigating the risks posed by volcanic eruptions. By combining data from these monitoring techniques, scientists can improve their ability to predict eruptions, issue timely warnings, and protect lives and property in volcanic regions.
Preparing for Volcanic Eruptions
Preparing for volcanic eruptions is essential for minimizing the impact on human lives, property, and infrastructure. Communities living in volcanic regions must have comprehensive emergency plans and evacuation procedures in place to ensure a swift and coordinated response in the event of an eruption.
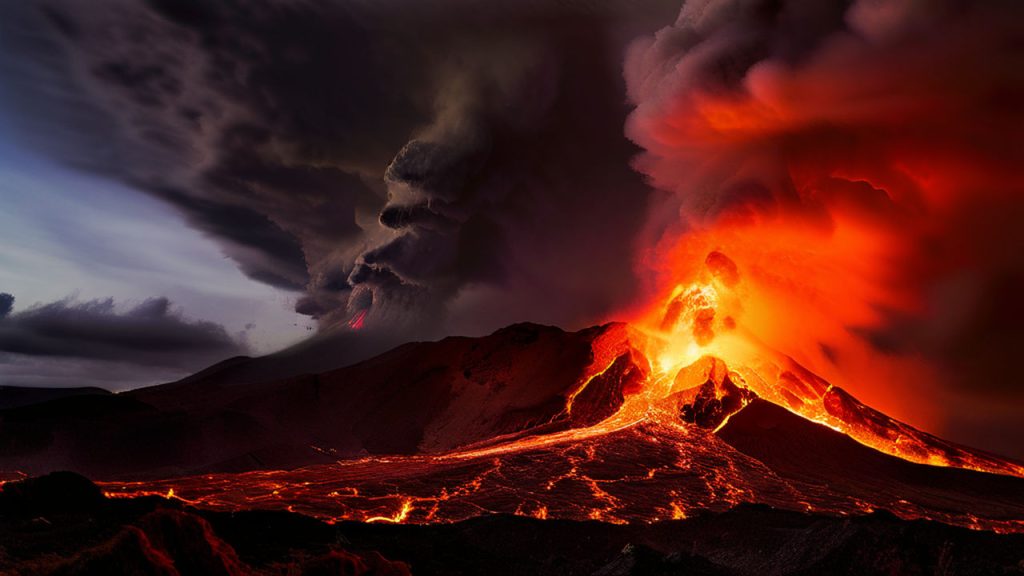
Emergency Plans
- Risk Assessment: Communities in volcanic regions should conduct thorough risk assessments to identify potential hazards associated with volcanic activity, such as lava flows, pyroclastic flows, lahars, ash fall, and volcanic gases. Understanding these hazards allows authorities to develop effective mitigation strategies and emergency plans.
- Communication Protocols: Establishing clear communication protocols is crucial for disseminating information and instructions to the public before, during, and after volcanic eruptions. Emergency plans should include methods for alerting residents about impending eruptions, evacuation orders, shelter locations, and safety precautions.
- Evacuation Routes: Identify and designate primary and secondary evacuation routes leading away from high-risk areas near the volcano. Ensure that these routes are well-marked, accessible, and capable of accommodating large volumes of traffic in the event of an evacuation. Considerations should also be made for individuals with mobility challenges and special needs.
- Emergency Shelters: Identify and designate emergency shelters located outside the hazard zone where evacuated residents can seek refuge during volcanic eruptions. These shelters should be equipped with essential supplies, such as food, water, medical supplies, and sanitation facilities, to accommodate evacuees for an extended period if necessary.
- Coordination with Authorities: Collaborate with local, regional, and national authorities, including emergency management agencies, law enforcement, medical services, and government officials, to coordinate response efforts and ensure a unified approach to disaster management. Establish protocols for activating emergency operations centers and deploying resources to affected areas.
Evacuation Procedures
- Early Warning Systems: Implement early warning systems, such as sirens, text alerts, social media notifications, and mobile apps, to alert residents about impending volcanic eruptions and evacuation orders. Ensure that warning messages are clear, concise, and accessible to all members of the community.
- Evacuation Orders: Issue evacuation orders promptly when volcanic activity poses an imminent threat to public safety. Clearly communicate evacuation instructions, including the evacuation route, assembly points, and shelter locations, to residents through multiple communication channels.
- Evacuation Assistance: Provide assistance to vulnerable populations, such as elderly individuals, people with disabilities, children, and those without transportation, to ensure they can evacuate safely. Mobilize community volunteers, emergency responders, and transportation services to assist with evacuation efforts and provide support to those in need.
- Traffic Management: Implement traffic management measures, such as lane reversals, road closures, and traffic control points, to facilitate the orderly evacuation of residents and prevent congestion on evacuation routes. Coordinate with law enforcement agencies to ensure smooth traffic flow and minimize delays.
- Post-Evacuation Support: Offer support and assistance to evacuated residents, including temporary housing, medical care, counseling services, and access to essential supplies. Keep evacuated residents informed about the latest developments, evacuation status, and when it is safe to return home through regular updates and communication channels.
In conclusion, preparing for volcanic eruptions requires proactive planning, effective communication, and coordinated response efforts to ensure the safety and well-being of communities at risk. By developing comprehensive emergency plans and evacuation procedures, authorities can mitigate the impact of volcanic disasters and protect lives and property in volcanic regions.
Case Studies
Mount St. Helens Eruption
The eruption of Mount St. Helens on May 18, 1980, is one of the most significant volcanic events in recent history. Located in Washington State, USA, Mount St. Helens is a stratovolcano known for its explosive eruptions and steep-sided cone.
Background:
Prior to the eruption, Mount St. Helens had been showing signs of increasing volcanic activity, including earthquake swarms, steam venting, and bulging of the volcano’s north flank. Despite these warning signs, the magnitude of the eruption took many by surprise.
Eruption Event:
On the morning of May 18, 1980, a massive landslide triggered by a magnitude 5.1 earthquake caused the north flank of Mount St. Helens to collapse, releasing pressure on the volcano’s magma chamber. This sudden release of pressure led to a lateral blast that devastated an area of over 600 square kilometers, flattening forests, melting glaciers, and sending a massive ash plume into the atmosphere.
Impacts:
The eruption of Mount St. Helens resulted in 57 fatalities and caused widespread destruction of infrastructure, including roads, bridges, and buildings. The ash plume rose to an altitude of over 20 kilometers and spread across the United States, disrupting air travel and affecting weather patterns. Lahars generated by the melting of glaciers inundated river valleys, further adding to the destruction.
Response and Recovery:
In the aftermath of the eruption, emergency responders, including the National Guard and search and rescue teams, conducted search and rescue operations to locate survivors and recover victims. Evacuation orders were issued for nearby communities, and emergency shelters were established to provide refuge for displaced residents.
Eyjafjallajökull Eruption
The eruption of Eyjafjallajökull in Iceland in April 2010 captured global attention due to its impact on air travel and international commerce. Eyjafjallajökull is a stratovolcano located beneath a glacier of the same name in southern Iceland.
Background:
The eruption of Eyjafjallajökull began on March 20, 2010, following a series of earthquakes beneath the volcano. The eruption initially produced lava flows and ash plumes, but the situation escalated on April 14 when a new vent opened beneath the glacier, causing a massive phreatomagmatic eruption.
Eruption Event:
The phreatomagmatic eruption beneath Eyjafjallajökull’s glacier resulted in the rapid melting of ice, leading to powerful explosions and the generation of large ash plumes. The eruption column reached heights of up to 9 kilometers, blanketing large areas of Europe with volcanic ash and disrupting air travel across the continent.
Impacts:
The eruption of Eyjafjallajökull caused widespread disruption to air travel, with thousands of flights canceled or diverted due to concerns about volcanic ash damaging aircraft engines. The economic impact of the eruption was significant, costing airlines billions of dollars in lost revenue and disrupting global supply chains.
Response and Recovery:
In response to the eruption, aviation authorities implemented flight restrictions and no-fly zones in affected areas to ensure the safety of air travelers. Governments and emergency management agencies provided support to affected communities, including assistance with cleanup efforts and the provision of emergency supplies.
Lessons Learned:
The eruptions of Mount St. Helens and Eyjafjallajökull underscore the importance of effective monitoring, preparedness, and response measures in mitigating the impacts of volcanic disasters. Both events serve as reminders of the need for ongoing research, public education, and collaboration among scientists, emergency responders, and policymakers to enhance resilience and reduce the risk posed by volcanic activity.
Environmental Impacts
Volcanic eruptions can have significant environmental impacts, affecting air quality, climate, and ecosystems both locally and globally. Understanding these impacts is crucial for assessing the long-term consequences of volcanic activity on the environment and implementing measures to mitigate its effects.
Air Quality
- Emission of Volcanic Gases: During volcanic eruptions, large quantities of gases, including sulfur dioxide (SO2), carbon dioxide (CO2), hydrogen sulfide (H2S), and hydrochloric acid (HCl), are released into the atmosphere. These gases can have detrimental effects on air quality, leading to respiratory problems, acid rain, and damage to vegetation.
- Formation of Volcanic Ash: Volcanic eruptions also produce volcanic ash, fine particles of pulverized rock and glass, which can remain suspended in the atmosphere for extended periods. Inhalation of volcanic ash can cause respiratory issues and exacerbate pre-existing respiratory conditions, such as asthma and bronchitis.
- Impact on Visibility: The presence of volcanic ash and gases in the atmosphere can reduce visibility and create hazardous conditions for air travel and transportation. Volcanic ash clouds can interfere with aircraft engines, leading to flight cancellations, diversions, and delays.
- Health Risks: Exposure to volcanic gases and ash can pose health risks to humans and animals, including irritation of the respiratory tract, eye irritation, and skin problems. Vulnerable populations, such as children, the elderly, and individuals with pre-existing health conditions, are particularly at risk of adverse health effects.
Climate Effects
- Injection of Aerosols into the Stratosphere: Volcanic eruptions inject large quantities of sulfur dioxide (SO2) and other aerosols into the stratosphere, where they can remain for months to years. These aerosols reflect incoming solar radiation back into space, leading to a temporary cooling effect on the Earth’s surface known as volcanic cooling.
- Impact on Global Temperature: The cooling effect of volcanic aerosols can offset the warming caused by greenhouse gas emissions, resulting in a temporary reduction in global temperatures. Major volcanic eruptions, such as the 1991 eruption of Mount Pinatubo, have been associated with short-term cooling periods lasting for one to two years.
- Disruption of Weather Patterns: Volcanic eruptions can disrupt atmospheric circulation patterns, leading to changes in weather patterns and precipitation regimes. The injection of aerosols into the stratosphere can alter the distribution of heat and moisture in the atmosphere, influencing regional climate variability.
- Effects on Agriculture and Ecosystems: Changes in climate resulting from volcanic eruptions can have implications for agriculture, forestry, and natural ecosystems. Shifts in temperature and precipitation patterns can affect crop yields, water availability, and biodiversity, leading to economic losses and environmental degradation.
In conclusion, volcanic eruptions can have significant environmental impacts, including degradation of air quality, disruption of climate patterns, and alteration of ecosystems. Monitoring and mitigating these impacts require interdisciplinary research, collaboration among scientists and policymakers, and proactive measures to enhance resilience and adaptability to volcanic activity.
Socio-economic Effects
Volcanic eruptions can have profound socio-economic effects, impacting communities, economies, and livelihoods in affected regions. Understanding these effects is essential for implementing effective disaster management strategies and supporting the recovery and resilience of affected populations.
Displacement of Communities
- Forced Evacuations: Volcanic eruptions can trigger forced evacuations of nearby communities to ensure the safety of residents in high-risk areas. Evacuated populations may experience disruption to their daily lives, loss of property, and separation from family members and social networks.
- Temporary Sheltering: Evacuated communities may require temporary sheltering in emergency shelters or evacuation centers until it is safe to return home. Providing adequate shelter, food, water, and medical care to displaced populations is essential for meeting their basic needs and ensuring their well-being during times of crisis.
- Long-term Displacement: In some cases, volcanic eruptions can lead to long-term displacement of communities if their homes and livelihoods are severely impacted by the disaster. Displaced populations may face challenges in finding permanent housing, employment, and access to essential services, prolonging their recovery and resettlement process.
- Psychosocial Impacts: The displacement of communities due to volcanic eruptions can have profound psychosocial impacts on individuals and families, including stress, anxiety, trauma, and grief. Providing mental health support and psychosocial services to affected populations is critical for addressing their emotional and psychological needs and promoting resilience and coping mechanisms.
Economic Repercussions
- Loss of Livelihoods: Volcanic eruptions can disrupt local economies by damaging agricultural lands, infrastructure, and businesses in affected areas. Displaced populations may lose their sources of income and livelihoods, exacerbating poverty and socio-economic inequality.
- Damage to Infrastructure: The destruction of infrastructure, such as roads, bridges, utilities, and buildings, can impede economic activities and hinder recovery efforts in volcanic-affected regions. Rebuilding and repairing damaged infrastructure require significant financial resources and coordination among government agencies, donors, and international organizations.
- Impact on Tourism: Volcanic eruptions can have adverse effects on tourism-dependent economies by disrupting travel, closing tourist attractions, and damaging natural landscapes and cultural heritage sites. The loss of tourist revenue can have ripple effects on local businesses, employment, and community development initiatives.
- Environmental Degradation: The environmental impacts of volcanic eruptions, such as air and water pollution, soil erosion, and habitat destruction, can have long-term consequences for ecosystem services and natural resources. Degraded environments may affect agriculture, fisheries, and other sectors dependent on healthy ecosystems, leading to economic losses and food insecurity.
In conclusion, the socio-economic effects of volcanic eruptions are complex and multifaceted, affecting communities, economies, and ecosystems in diverse ways. Addressing these effects requires comprehensive disaster management strategies, including preparedness, response, recovery, and resilience-building efforts, to support the well-being and livelihoods of affected populations and promote sustainable development in volcanic-affected regions.
Conclusion
In conclusion, volcanic eruptions are complex natural phenomena that can have significant impacts on the environment, communities, and economies. Understanding the mechanisms of volcanic eruptions, including magma formation, triggering mechanisms, and eruption styles, is essential for assessing the potential hazards and risks associated with volcanic activity.
Throughout this article, we have explored the various factors that contribute to volcanic eruptions, from the geological processes shaping Earth’s structure to the socio-economic effects on human populations. We have discussed the importance of monitoring volcanic activity using techniques such as seismographs, gas emission measurements, and ground deformation monitoring to predict eruptions and mitigate their impacts.
We have also examined the socio-economic effects of volcanic eruptions, including the displacement of communities and the economic repercussions on local economies. Displaced populations may face challenges in finding shelter, employment, and access to essential services, highlighting the importance of comprehensive disaster management strategies and support systems for affected communities.
In light of these challenges, preparedness and understanding play crucial roles in mitigating the impacts of volcanic eruptions. By developing emergency plans, evacuation procedures, and early warning systems, communities can minimize the risks to human lives and property and enhance their resilience to volcanic disasters.
Furthermore, fostering a deeper understanding of volcanic processes and their potential impacts among policymakers, scientists, and the general public is essential for effective decision-making and risk management. By working together to enhance preparedness, response, and recovery efforts, we can reduce the vulnerability of communities to volcanic hazards and build more resilient societies in volcanic-affected regions.
In conclusion, the study of volcanic eruptions is not only a scientific endeavor but also a critical component of disaster risk reduction and sustainable development efforts worldwide. By acknowledging the complexities of volcanic systems and their interactions with human societies and the environment, we can better prepare for and respond to the challenges posed by volcanic activity and promote safety, resilience, and well-being for all.
FAQs
- What causes volcanic eruptions?
Volcanic eruptions are primarily caused by the movement of magma from within the Earth’s mantle towards the surface. This movement can be triggered by factors such as tectonic activity, pressure buildup, and the infiltration of water into volcanic systems. - How can volcanic eruptions be predicted?
Volcanic eruptions can be predicted through monitoring techniques such as seismology, gas emission measurements, and ground deformation monitoring. Changes in seismic activity, gas emissions, and ground deformation patterns can provide valuable insights into the likelihood and timing of volcanic eruptions. - What are the different types of volcanic eruptions?
There are several types of volcanic eruptions, including effusive eruptions, explosive eruptions, and phreatic eruptions. Effusive eruptions involve the gentle outpouring of lava, while explosive eruptions are characterized by violent explosions of ash, rock fragments, and volcanic gases. Phreatic eruptions occur when groundwater comes into contact with hot volcanic rocks, leading to steam-driven explosions. - How do volcanic eruptions impact the environment?
Volcanic eruptions can have significant environmental impacts, including degradation of air quality, disruption of climate patterns, and alteration of ecosystems. The emission of volcanic gases and ash can lead to respiratory problems, acid rain, and damage to vegetation, while the injection of aerosols into the atmosphere can cause temporary cooling of the Earth’s surface. - What should I do in the event of a volcanic eruption?
In the event of a volcanic eruption, it is important to follow the instructions of local authorities and emergency responders. If you are in a high-risk area, evacuate to a safe location following designated evacuation routes. Stay informed about the latest developments and alerts through official communication channels, and be prepared to take appropriate safety precautions to protect yourself and your loved ones.
No Comments
Leave a comment Cancel