Certainly! Let’s expand on the introduction to provide more context and engage the reader further.
Introduction
Welcome to the captivating world of photosynthesis! In this article, we embark on a journey into the heart of one of nature’s most extraordinary phenomena – the conversion of sunlight into life-sustaining energy. As we unravel the intricacies of photosynthesis, we will uncover the remarkable mechanisms employed by plants, algae, and certain bacteria to thrive in their environments.
Imagine a lush green forest bathed in the golden glow of sunlight, or a vibrant coral reef teeming with life beneath the azure waves of the ocean. Behind these breathtaking scenes lies a fundamental process that powers the entire ecosystem – photosynthesis. It is the engine that drives the growth of towering trees, the bloom of colorful flowers, and the proliferation of microscopic organisms that form the basis of the food chain.
But photosynthesis is much more than just a biological function; it is a masterpiece of molecular choreography, meticulously orchestrated within the confines of tiny cellular factories known as chloroplasts. Here, sunlight is captured, water is split, and carbon dioxide is transformed into the building blocks of life. It is a symphony of chemical reactions, each step finely tuned to extract the maximum energy from the sun’s rays.
As we delve deeper into the inner workings of photosynthesis, we will unravel its mysteries and appreciate its profound significance for life on Earth. From the oxygen we breathe to the food we eat, photosynthesis touches every aspect of our existence, weaving a delicate tapestry of interconnectedness that binds all living organisms together.
So join us on this illuminating exploration of photosynthesis, where science meets poetry, and the wonders of the natural world unfold before our eyes. Together, let us marvel at the ingenuity of evolution and the beauty of the planet we call home.
What is Photosynthesis?
Photosynthesis is a complex biochemical process utilized by green plants, algae, and certain bacteria to convert sunlight, carbon dioxide (CO2), and water (H2O) into organic compounds, predominantly glucose, and oxygen (O2). This intricate process plays a pivotal role in sustaining life on our planet by serving as the primary source of oxygen production and forming the basis of the food chain.
At its core, photosynthesis involves the capture and transformation of light energy into chemical energy, which is then used to power various metabolic reactions within the organism. The process takes place within specialized cellular structures called chloroplasts, where chlorophyll, a green pigment, absorbs sunlight and initiates the series of reactions that ultimately result in the synthesis of glucose and the release of oxygen.
By harnessing the energy of sunlight, plants and other photosynthetic organisms are able to convert carbon dioxide from the atmosphere and water from the soil into carbohydrates, such as glucose. These carbohydrates serve as the primary source of energy for the organism’s growth and metabolic functions.
Moreover, photosynthesis plays a crucial role in maintaining the balance of atmospheric gases. As plants photosynthesize, they release oxygen as a byproduct, which is essential for the respiration of organisms, including humans. Additionally, photosynthesis helps regulate the levels of carbon dioxide in the atmosphere, serving as a natural mechanism for mitigating climate change by absorbing excess CO2 from the air.
In essence, photosynthesis is not only a fundamental process for the survival of plants and other photosynthetic organisms but also a cornerstone of Earth’s ecosystems. Its ability to harness solar energy and produce oxygen makes it one of the most significant biochemical reactions on our planet, shaping the conditions necessary for life to flourish.
The Role of Chloroplasts
Chloroplasts are specialized organelles found in the cells of plants and some other photosynthetic organisms, where the process of photosynthesis takes place. These remarkable structures are responsible for capturing light energy from the sun and converting it into chemical energy that can be used by the cell.
Within the chloroplasts, a green pigment called chlorophyll plays a central role in the absorption of light energy. Chlorophyll molecules are located within the thylakoid membranes, which are arranged in stacks called grana. This arrangement maximizes the surface area available for light absorption and facilitates the transfer of energy to the photosynthetic machinery.
As sunlight strikes the chlorophyll molecules, they absorb photons of light energy, initiating a series of chemical reactions that ultimately result in the conversion of carbon dioxide and water into glucose and oxygen. This process occurs in two main stages: the light-dependent reactions and the light-independent reactions (Calvin cycle), both of which take place within the chloroplasts.
In addition to chlorophyll, chloroplasts contain other pigments and proteins that are essential for the photosynthetic process. These include carotenoids, which help broaden the range of light wavelengths that can be absorbed, and various enzymes and electron carriers involved in the synthesis of ATP and NADPH, which are energy-rich molecules used to drive the synthesis of carbohydrates.
Furthermore, chloroplasts are dynamic organelles capable of adjusting their structure and function in response to environmental cues. For example, changes in light intensity or availability can trigger alterations in the distribution and activity of chloroplasts within the cell, optimizing photosynthetic efficiency and ensuring the plant’s survival.
Overall, chloroplasts play a crucial role in the process of photosynthesis, serving as the primary sites where light energy is captured and converted into chemical energy. Without these specialized organelles, plants would be unable to harness the energy of sunlight and produce the essential carbohydrates and oxygen necessary for their growth and survival.
Structure of Chlorophyll
Chlorophyll molecules possess a unique structure consisting of a porphyrin ring with a magnesium ion at its center. This distinctive arrangement enables chlorophyll to efficiently absorb light energy, particularly in the blue and red regions of the electromagnetic spectrum.
The porphyrin ring, which serves as the core structure of chlorophyll, is composed of four nitrogen atoms linked by carbon atoms. At the center of this ring lies a magnesium ion, which plays a crucial role in the molecule’s ability to capture and transfer light energy.
When sunlight strikes a chlorophyll molecule, photons of light are absorbed by the magnesium ion at the center of the porphyrin ring. This absorption of light energy triggers a series of chemical reactions within the chlorophyll molecule, leading to the initiation of photosynthesis.
Interestingly, chlorophyll molecules are selective in the wavelengths of light they absorb and reflect. While chlorophyll efficiently absorbs light in the blue and red regions of the spectrum, it reflects green light, giving plants their characteristic green coloration. This phenomenon is why plants appear green to the human eye, as green light is not absorbed but rather reflected by chlorophyll molecules.
By harnessing the energy of absorbed light, chlorophyll molecules drive the process of photosynthesis, ultimately converting carbon dioxide and water into glucose and oxygen. This remarkable ability to capture and utilize light energy lies at the heart of plant growth and sustenance, making chlorophyll a crucial component of the photosynthetic machinery.
In summary, the unique structure of chlorophyll, with its porphyrin ring and central magnesium ion, enables plants to capture and convert light energy into chemical energy, fueling the process of photosynthesis and supporting life on Earth.
The Two Stages of Photosynthesis
Photosynthesis is a complex process that can be divided into two main stages: the light-dependent reactions and the light-independent reactions, also known as the Calvin cycle. These two stages work in concert to convert light energy into chemical energy, ultimately producing glucose and oxygen.
Light-Dependent Reactions
The first stage of photosynthesis, known as the light-dependent reactions, takes place in the thylakoid membranes of the chloroplasts. This stage requires light energy to drive a series of complex chemical reactions.
During the light-dependent reactions:
- Absorption of Light: Chlorophyll molecules within the thylakoid membranes absorb photons of light energy.
- Water Splitting: This absorbed light energy is used to split water molecules into oxygen, protons (H+ ions), and electrons (e-).
- Generation of ATP and NADPH: The energy from the absorbed light is utilized to generate two crucial energy-carrying molecules: adenosine triphosphate (ATP) and nicotinamide adenine dinucleotide phosphate (NADPH). These molecules serve as the primary sources of energy for the subsequent reactions in photosynthesis.
Calvin Cycle (Light-Independent Reactions)
The second stage of photosynthesis, the Calvin cycle, occurs in the stroma of the chloroplasts and does not directly require light. Instead, it relies on the products of the light-dependent reactions, ATP, and NADPH, to drive the synthesis of glucose from carbon dioxide.
During the Calvin cycle:
- Carbon Fixation: Carbon dioxide from the atmosphere is captured and converted into a stable organic molecule, known as ribulose-1,5-bisphosphate (RuBP), with the help of the enzyme RuBisCO.
- Reduction Phase: ATP and NADPH produced in the light-dependent reactions are utilized to power the conversion of carbon dioxide into glucose. This involves a series of enzymatic reactions that result in the formation of carbohydrates.
- Regeneration of RuBP: The remaining molecules in the cycle are rearranged and regenerated to sustain the continuous flow of carbon through the cycle.
Factors Affecting Photosynthesis
The rate of photosynthesis, the process by which plants convert light energy into chemical energy, is influenced by various factors that affect the availability of light, carbon dioxide, and water, as well as the temperature and the presence of certain enzymes.
Light Intensity
The intensity of light plays a critical role in influencing the rate of photosynthesis in plants. Higher light intensity generally leads to increased photosynthetic activity, as more photons are available to drive the light-dependent reactions of photosynthesis. This means that plants exposed to ample sunlight are typically able to photosynthesize at a faster rate compared to those in shaded or low-light environments.
However, it’s important to note that the relationship between light intensity and photosynthetic rate is not linear. As light intensity continues to increase, there comes a point where the rate of photosynthesis reaches a maximum level, and further increases in light intensity do not lead to corresponding increases in photosynthetic activity. This phenomenon is known as saturation.
At saturation, the photosynthetic machinery of the plant becomes fully activated, and additional photons of light are not effectively utilized. Instead, other factors such as the availability of carbon dioxide or the capacity of the plant’s photosynthetic enzymes may become limiting factors.
In natural environments, light intensity can vary throughout the day due to factors such as cloud cover, shade from nearby vegetation, or changes in the angle of sunlight. Plants have evolved mechanisms to adapt to these fluctuations in light intensity, adjusting their photosynthetic activity accordingly.
In agricultural settings, optimizing light intensity is crucial for maximizing crop yields. Techniques such as providing supplemental lighting in greenhouses or adjusting planting densities to ensure adequate light exposure can help enhance photosynthetic efficiency and improve overall productivity.
In summary, while higher light intensity generally promotes increased photosynthetic activity in plants, there is a point of saturation where further increases in light intensity do not lead to proportional increases in photosynthesis. Understanding and managing light intensity is essential for optimizing plant growth and productivity in both natural and controlled environments.
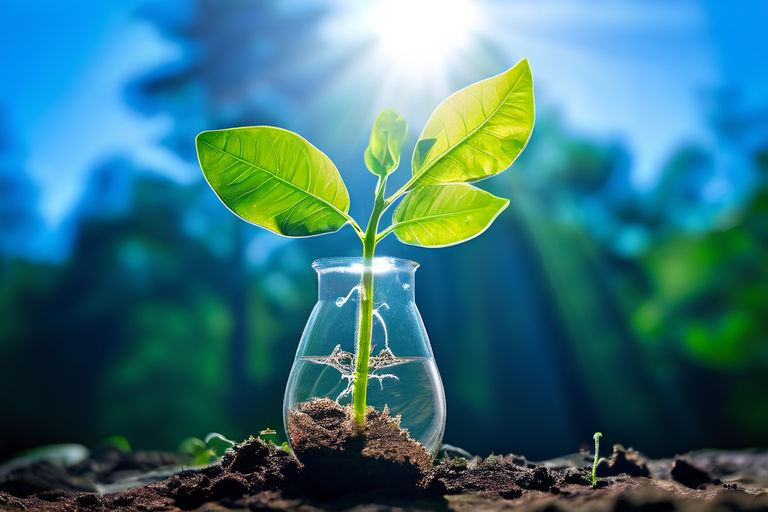
Carbon Dioxide Concentration
The concentration of carbon dioxide (CO2) in the surrounding environment significantly influences the rate of photosynthesis in plants. Elevated levels of CO2 can enhance photosynthetic activity by providing plants with more carbon dioxide molecules to incorporate into organic compounds through the Calvin cycle, the light-independent reactions of photosynthesis.
As CO2 concentration increases, the rate of photosynthesis generally rises, leading to increased production of glucose and other carbohydrates. This phenomenon is particularly evident in environments where CO2 levels are artificially elevated, such as in controlled greenhouse settings or under conditions of increased carbon dioxide emissions.
However, it’s essential to recognize that the relationship between CO2 concentration and photosynthetic rate is not linear. While higher CO2 levels initially stimulate photosynthesis, there comes a point where the rate of photosynthesis reaches a plateau, and further increases in CO2 concentration do not lead to additional gains in photosynthetic activity. At this point, other factors such as light intensity, temperature, or the availability of water may become limiting factors for photosynthesis.
This plateau effect occurs because photosynthesis is a complex biochemical process that depends on the coordinated interaction of multiple factors. Once CO2 levels reach a certain threshold, the rate of photosynthesis may be limited by other factors rather than the availability of carbon dioxide.
Understanding the response of plants to varying CO2 concentrations is crucial for predicting how ecosystems will respond to changes in atmospheric CO2 levels, such as those associated with climate change. Elevated CO2 concentrations can have profound effects on plant growth, ecosystem dynamics, and global carbon cycling, with potential implications for agriculture, forestry, and biodiversity conservation.
In summary, while elevated CO2 levels generally enhance photosynthesis in plants, there is a point of saturation where further increases in CO2 concentration do not lead to proportional increases in photosynthetic activity. Consideration of other environmental factors is essential for understanding the complex dynamics of photosynthesis and its implications for ecosystems and the global carbon cycle.
Temperature
Temperature plays a crucial role in influencing the rate of photosynthesis in plants. Optimal temperatures promote enzyme activity and photosynthetic efficiency, facilitating the biochemical reactions necessary for the synthesis of carbohydrates from carbon dioxide and water.
In general, photosynthesis occurs most efficiently within a specific temperature range that varies among plant species. This optimal temperature range is typically between 15°C to 35°C (59°F to 95°F), although it may vary depending on factors such as the plant’s evolutionary history and environmental adaptations.
At optimal temperatures:
- Enzymes involved in photosynthesis exhibit maximum activity, allowing for efficient catalysis of biochemical reactions. These enzymes include ribulose-1,5-bisphosphate carboxylase/oxygenase (RuBisCO), which is essential for carbon fixation in the Calvin cycle.
- Photosynthetic pigments, such as chlorophyll, function optimally, enabling effective absorption of light energy.
- Metabolic processes within the chloroplasts proceed smoothly, ensuring the continuous production of ATP and NADPH, which are essential energy carriers for photosynthesis.
However, extreme temperatures can disrupt the delicate balance of photosynthetic processes, leading to reduced photosynthetic efficiency and impaired plant growth. High temperatures can accelerate the rate of enzyme denaturation, causing the loss of enzyme activity and inhibiting photosynthetic reactions. Conversely, low temperatures can slow down metabolic reactions and limit the availability of energy for photosynthesis.
At extreme temperatures:
- Enzymes may undergo denaturation, resulting in the loss of their three-dimensional structure and functional activity. This can severely impair the functioning of key enzymes involved in photosynthesis, such as RuBisCO.
- Membrane integrity may be compromised, leading to leakage of cellular contents and disruption of ion gradients essential for photosynthetic processes.
- Photosynthetic pigments may become damaged or degraded, reducing their ability to absorb light energy effectively.
As a result, plants exposed to extreme temperatures may experience reduced rates of photosynthesis, decreased growth, and increased susceptibility to environmental stressors.
In agricultural settings, managing temperature is essential for optimizing crop productivity and resilience to environmental fluctuations. Techniques such as providing shade, irrigation, and thermal insulation can help mitigate the effects of extreme temperatures on photosynthesis and plant health.
In summary, while optimal temperatures promote enzyme activity and photosynthetic efficiency, extreme temperatures can have detrimental effects on photosynthesis by disrupting biochemical processes and damaging cellular structures. Understanding the temperature requirements of plants is essential for maximizing crop yields and maintaining ecosystem health.
Conclusion
In conclusion, photosynthesis stands as a remarkable biological process that serves as the cornerstone of life on Earth. Through the intricate conversion of light energy into organic compounds and oxygen, photosynthesis sustains the diverse array of ecosystems that populate our planet.
This process not only fuels the growth and development of plants but also plays a vital role in maintaining the balance of atmospheric gases, providing the oxygen necessary for respiration and supporting the intricate web of life that inhabits our world.
By delving into the intricacies of photosynthesis, we gain a deeper understanding of the interconnectedness of all living organisms and the delicate balance that sustains life on Earth. From the towering trees of dense forests to the microscopic algae in vast oceans, every organism is intricately linked by the fundamental process of photosynthesis.
As we marvel at the ingenuity of nature’s design, let us also recognize the profound implications of photosynthesis for our own existence. From the air we breathe to the food we eat, the impact of photosynthesis reverberates throughout every aspect of our lives, reminding us of the remarkable symbiosis between humanity and the natural world.
In understanding and appreciating the intricacies of photosynthesis, we gain a newfound reverence for the beauty and complexity of the natural world. It is a testament to the power of life to adapt, evolve, and thrive in even the harshest of environments, reminding us of the resilience and interconnectedness that define our planet.
As stewards of this planet, it is our responsibility to safeguard and preserve the delicate balance of life that photosynthesis sustains. By embracing sustainable practices and fostering a deeper connection to the natural world, we can ensure a brighter and more vibrant future for generations to come.
FAQs (Frequently Asked Questions)
1. Why is photosynthesis important? Photosynthesis is crucial as it provides oxygen for respiration and serves as the foundation of the food chain, sustaining life on Earth.
2. What happens to the oxygen produced during photosynthesis? The oxygen released during photosynthesis is either used for respiration by plants and other organisms or released into the atmosphere.
3. Can photosynthesis occur without sunlight? Photosynthesis relies on light energy to drive the process; hence, it cannot occur in the absence of sunlight.
4. How does photosynthesis impact the environment? Photosynthesis helps mitigate climate change by absorbing carbon dioxide from the atmosphere and producing oxygen.
5. Are there organisms that do not undergo photosynthesis? Yes, certain organisms, such as fungi and most animals, do not perform photosynthesis and obtain their energy by consuming organic matter.
No Comments
Leave a comment Cancel