Introduction to Genetic Engineering
Genetic engineering, a revolutionary field in biotechnology, holds immense potential in altering the genetic makeup of organisms. Through precise manipulation of DNA, scientists can introduce new traits, modify existing ones, or even create entirely new organisms. This article delves into the intricate process of genetic engineering, exploring its techniques, applications, and ethical considerations.
Fundamentals of Genetic Engineering
- DNA Isolation and Manipulation: The initial step in genetic engineering involves isolating the DNA of interest from the organism’s genome and manipulating it in a controlled environment. Techniques such as polymerase chain reaction (PCR) and restriction enzyme digestion facilitate the isolation and amplification of specific DNA sequences.
- Gene Cloning: Gene cloning enables the replication of DNA fragments by inserting them into vectors, such as plasmids or viral genomes, which act as carriers to transfer the DNA into host organisms, typically bacteria or yeast. This process generates multiple copies of the target gene for further analysis or manipulation.
- Gene Editing Technologies: Recent advancements in gene editing technologies, such as CRISPR-Cas9, Zinc Finger Nucleases (ZFNs), and Transcription Activator-Like Effector Nucleases (TALENs), have revolutionized genetic engineering by allowing precise modification of DNA sequences. These tools enable scientists to edit genes with unprecedented accuracy, offering immense potential for both research and practical applications.
These fundamental techniques form the cornerstone of genetic engineering, providing scientists with the tools and methodologies to manipulate genetic material for various purposes, ranging from basic research to the development of novel therapies and biotechnological solutions.
Techniques in Genetic Engineering
- Recombinant DNA Technology: Recombinant DNA technology involves the manipulation of DNA molecules from different sources to create novel combinations of genetic material. This technique allows scientists to insert specific genes into host organisms, facilitating the production of desired proteins or the modification of genetic traits.
- CRISPR-Cas9: CRISPR-Cas9 is a revolutionary gene editing tool derived from bacterial immune systems. It utilizes RNA molecules to guide the Cas9 enzyme to specific target sequences in DNA, where it induces precise changes or modifications. CRISPR-Cas9 offers unparalleled precision and efficiency in genome editing, making it a widely adopted technique in genetic engineering research and applications.
- Zinc Finger Nucleases (ZFNs): Zinc Finger Nucleases are engineered proteins designed to target and cleave specific DNA sequences. These proteins consist of zinc finger domains, which bind to target DNA sequences, fused with a nuclease domain that introduces double-strand breaks in the DNA. ZFNs have been used for targeted genome editing in a variety of organisms, although their use has been overshadowed by the emergence of CRISPR-Cas9 due to its simplicity and versatility.
- TALENs (Transcription Activator-Like Effector Nucleases): TALENs are another type of engineered nuclease used for targeted genome editing. Similar to ZFNs, TALENs are designed to bind to specific DNA sequences and induce double-strand breaks, enabling precise modifications of the genetic code. While TALENs offer high specificity and efficiency, they require more complex design and synthesis compared to CRISPR-Cas9.
These techniques represent the cutting-edge tools in genetic engineering, empowering scientists to manipulate DNA with unprecedented precision and efficiency. Each technique has its advantages and applications, contributing to the diverse toolkit available for genetic manipulation and research.
Applications of Genetic Engineering
- Agriculture: Genetic engineering has revolutionized agriculture by enabling the development of genetically modified (GM) crops with desirable traits, such as increased resistance to pests, diseases, and environmental stresses, as well as improved nutritional content and yield. GM crops have contributed to enhanced food security, reduced reliance on chemical pesticides, and sustainable agricultural practices.
- Medicine: In the field of medicine, genetic engineering holds promise for diagnosing, treating, and preventing a wide range of diseases and disorders. It encompasses gene therapy, where defective genes are corrected or replaced to treat genetic disorders, as well as the production of recombinant proteins and vaccines for therapeutic purposes. Genetic engineering also plays a crucial role in pharmacogenomics, personalized medicine, and regenerative medicine.
- Industrial Biotechnology: Genetic engineering is instrumental in industrial biotechnology, where microorganisms are engineered to produce valuable chemicals, enzymes, pharmaceuticals, and biofuels. Through metabolic engineering and synthetic biology approaches, microbial hosts are optimized for enhanced production efficiency, substrate utilization, and product yields, driving innovation in bioprocessing and sustainable manufacturing.
- Environmental Conservation: Genetic engineering offers innovative solutions for environmental conservation and remediation by harnessing the natural capabilities of microorganisms and plants. Applications include bioremediation, where genetically engineered organisms are deployed to degrade pollutants and contaminants in soil, water, and air. Additionally, genetic engineering contributes to the development of bio-based materials, renewable energy sources, and climate-resilient crops to mitigate environmental challenges and promote ecological sustainability.
These diverse applications underscore the transformative potential of genetic engineering across various sectors, addressing critical challenges and driving advancements in agriculture, medicine, industry, and environmental stewardship. As technology continues to evolve, genetic engineering is poised to play an increasingly pivotal role in shaping the future of science and society.
Ethical Considerations in Genetic Engineering
- Potential Risks: Genetic engineering raises concerns regarding potential risks to human health, ecosystems, and biodiversity. These risks include unintended consequences of genetic modifications, such as the creation of new allergens or toxins, the spread of genetically modified organisms (GMOs) into natural environments, and disruptions to ecological balances. Ethical deliberations must weigh the benefits of genetic engineering against its potential risks and uncertainties, ensuring responsible and informed decision-making.
- Genetic Privacy: The advent of genetic engineering technologies raises significant concerns about genetic privacy and data security. As genetic information becomes increasingly accessible through genome sequencing and genetic testing, individuals face risks of genetic discrimination, stigmatization, and unauthorized access to their genetic data. Safeguarding genetic privacy requires robust regulations, informed consent procedures, and transparent policies to protect individuals’ autonomy, confidentiality, and rights to genetic information.
- Socioeconomic Implications: Genetic engineering has profound socioeconomic implications, shaping access to healthcare, food security, and economic opportunities. Concerns arise regarding disparities in access to genetic technologies, healthcare disparities, and the commodification of life. Ethical considerations encompass issues of social justice, equity, and inclusivity, ensuring that genetic engineering benefits all segments of society and fosters equitable distribution of its benefits and burdens.
Navigating these ethical considerations in genetic engineering necessitates interdisciplinary dialogue, stakeholder engagement, and ethical frameworks that integrate scientific, social, cultural, and ethical perspectives. By fostering transparency, accountability, and public participation, ethical governance frameworks can promote responsible innovation, uphold ethical principles, and address societal concerns in the application of genetic engineering technologies.
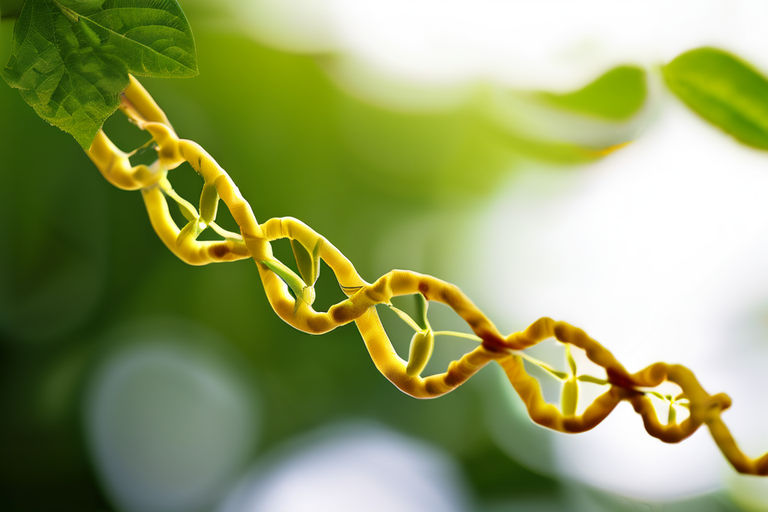
Challenges and Future Perspectives
- Regulatory Frameworks: Establishing robust regulatory frameworks is essential to govern the ethical and safe application of genetic engineering technologies. Regulatory bodies face challenges in keeping pace with rapid advancements in genetic engineering, ensuring risk assessment, monitoring, and oversight of research, development, and commercialization activities. Future perspectives involve harmonizing international regulations, promoting transparency, and engaging stakeholders to foster responsible innovation and ensure public trust in genetic engineering.
- Public Perception: Public perception plays a pivotal role in shaping the acceptance and adoption of genetic engineering technologies. Challenges arise from varying levels of understanding, misinformation, and ethical concerns among the public. Future perspectives entail fostering science communication, public engagement, and education initiatives to enhance awareness, address misconceptions, and build trust in the benefits and risks of genetic engineering. By promoting inclusive dialogue and participatory decision-making, stakeholders can bridge the gap between scientific advancements and societal values, fostering informed public discourse and acceptance of genetic engineering.
- Advancements in Precision Editing: The continual advancements in precision editing technologies, such as CRISPR-Cas9, present both opportunities and challenges in genetic engineering. While these tools offer unprecedented precision, efficiency, and versatility in genome editing, they also raise concerns about off-target effects, unintended consequences, and ethical implications. Future perspectives involve refining editing techniques, enhancing specificity, and developing safeguards to minimize risks and ensure the responsible application of precision editing in research, medicine, and biotechnology. By balancing scientific innovation with ethical considerations, stakeholders can harness the full potential of precision editing while mitigating potential harms and upholding societal values.
Genetic engineering represents a transformative frontier in scientific inquiry and technological innovation, heralding promising opportunities for addressing pressing challenges in health, agriculture, industry, and the environment. However, navigating the complexities of genetic engineering requires collective responsibility, ethical stewardship, and interdisciplinary collaboration to ensure that scientific progress aligns with ethical principles and societal values, promoting the common good and sustainable development.
Conclusion
In conclusion, genetic engineering stands as a testament to human ingenuity, offering unprecedented opportunities to harness the potential of DNA for the advancement of society. Through precise manipulation of genetic material, scientists have unlocked new avenues for innovation in agriculture, medicine, industry, and environmental conservation. However, the transformative power of genetic engineering also brings forth profound ethical considerations that demand careful deliberation and responsible action.
As we navigate the complexities of genetic engineering, it is imperative to foster dialogue, collaboration, and transparency among scientists, policymakers, and the public. By engaging in open discourse and ethical stewardship, we can ensure that scientific progress aligns with societal values and addresses the needs of present and future generations. Together, we can harness the power of genetic engineering for the greater good while mitigating potential risks and upholding ethical principles.
Frequently Asked Questions (FAQs)
- Is genetic engineering safe for the environment? Genetic engineering poses potential environmental risks, such as the unintended spread of genetically modified organisms (GMOs) and disruption of ecosystems. Rigorous testing and regulation are crucial to mitigate these concerns.
- Can genetic engineering be used to cure genetic diseases? Yes, genetic engineering holds promise in treating genetic diseases by correcting or replacing defective genes. However, ethical considerations and safety concerns must be carefully addressed in clinical applications.
- How does CRISPR-Cas9 work in genetic engineering? CRISPR-Cas9 is a precise gene-editing tool derived from bacterial immune systems. It utilizes RNA molecules to guide the Cas9 enzyme to specific target sequences in DNA, where it induces precise changes or modifications.
- What are the potential ethical implications of genetic engineering in humans? Ethical considerations in human genetic engineering include concerns about genetic discrimination, eugenics, and the commodification of life. Balancing scientific advancement with ethical principles is essential to navigate these complex issues.
- How can the public engage in discussions about genetic engineering? The public can participate in discussions about genetic engineering through education, advocacy, and involvement in policy-making processes. Promoting transparency and inclusivity in decision-making is vital for ensuring that genetic engineering serves the common good.