Introduction
Understanding the fundamental nature of matter is akin to unraveling the mysteries of the universe itself. From the solidity of a rock to the ethereal dance of a flame, matter manifests in diverse forms, each with its own set of properties and behaviors. In this exploration, we embark on a journey through the various states of matter, from the familiar solidity of a rock to the enigmatic realm of plasma and beyond. Through this journey, we will delve into the intricate interplay of particles and forces that govern the behavior of matter, shedding light on its essence and significance in the grand tapestry of existence. Join us as we embark on an odyssey through the cosmos of matter, where the boundaries of the known dissolve, and the wonders of the unknown await.
Understanding Matter
Matter, in its myriad forms, constitutes the very fabric of our universe. It encompasses everything tangible and intangible, from the air we breathe to the stars that adorn the night sky. Understanding the nature of matter is paramount to comprehending the complexities of our surroundings and the underlying principles that govern them.
Defining Matter
At its core, matter can be defined as anything that occupies space and possesses mass. It is the building block of all physical objects, ranging from the infinitesimally small subatomic particles to the vast celestial bodies that populate the cosmos.
Properties of Matter
Matter exhibits a diverse array of properties, each offering valuable insights into its behavior and interactions. These properties include mass, volume, density, and various others, which play pivotal roles in shaping the characteristics of different forms of matter. Understanding these properties is essential for unraveling the mysteries of the universe and harnessing the power of matter for technological advancements and scientific exploration.
The Three Fundamental States of Matter
The universe is teeming with matter, existing in a variety of states, each with distinct properties and behaviors. Among these, three fundamental states stand out: solid, liquid, and gas. Let’s delve into each of these states and explore their unique characteristics.
Solid State
Solid matter is characterized by a fixed shape and volume, owing to the close proximity and strong bonds between its constituent particles. In solids, the particles vibrate in fixed positions, maintaining a stable structure.
Properties of Solids
- Definite Shape: Solids retain a specific shape under normal conditions, as the arrangement of particles remains relatively fixed.
- Definite Volume: Similarly, solids have a fixed volume, as the particles are tightly packed together.
- High Density: The particles in solids are densely packed, resulting in a high density compared to liquids and gases.
- Limited Compressibility: Due to the close arrangement of particles, solids are generally difficult to compress.
Examples of Solids
Solid matter manifests in a myriad of forms, ranging from natural minerals to human-made materials. Common examples of solids include metals such as iron and copper, minerals like quartz and diamond, as well as everyday objects like wood and plastic.
Liquid State
In the vast expanse of the universe, matter exhibits a remarkable diversity of states, with liquids standing as one of the fundamental forms. Unlike solids, which maintain a fixed shape, liquids possess the ability to flow and adapt to the shape of their container, making them highly adaptable and versatile.
Properties of Liquids
Liquids boast a set of distinct properties that distinguish them from solids and gases:
- Indefinite Shape: Unlike solids, liquids do not have a fixed shape and instead conform to the shape of their container, allowing them to flow freely.
- Definite Volume: Despite their ability to change shape, liquids maintain a constant volume, as the particles are closely packed but still able to move past one another.
- Moderate Density: Liquids typically exhibit a moderate density, falling between the high density of solids and the low density of gases.
- Moderate Compressibility: While liquids are more compressible than solids, they are less compressible than gases, owing to the relatively close arrangement of particles.
Examples of Liquids
Liquids manifest in a myriad of forms across the universe, ranging from essential substances like water to complex compounds and solutions. Some common examples of liquids include:
- Water: The most abundant liquid on Earth, essential for life and existing in various forms such as liquid water, ice, and vapor.
- Oil: Various types of oils, including vegetable oil, mineral oil, and petroleum-based oils, serve diverse purposes ranging from cooking to lubrication.
- Alcohol: Ethanol and other alcoholic beverages represent another category of liquids, prized for their recreational and industrial uses.
- Mercury: A unique liquid metal, mercury exhibits unusual properties such as high density and low surface tension, making it valuable in scientific instruments and industrial applications.
Gaseous State
In the vast expanse of the cosmos, matter manifests in various states, with gases representing one of the fundamental forms. Unlike solids and liquids, gases lack a fixed shape and volume, instead, they expand to fill the entirety of their container, exhibiting remarkable flexibility and mobility.
Properties of Gases
Gases possess a distinctive set of properties that differentiate them from solids and liquids:
- Indefinite Shape and Volume: Gases have neither a fixed shape nor volume, as they uniformly fill the container they occupy.
- Low Density: Compared to solids and liquids, gases typically exhibit a low density, with particles being widely spaced apart.
- High Compressibility: Gas particles are highly compressible, allowing them to be squeezed into a smaller volume under pressure.
- Diffusion and Effusion: Gases diffuse rapidly through space and effuse through small openings due to the constant random motion of their particles.
Examples of Gases
Gaseous substances permeate the universe, from the atmosphere surrounding our planet to the distant reaches of interstellar space. Here are some common examples of gases:
- Oxygen (O<sub>2</sub>): Essential for respiration and combustion, oxygen gas is abundant in the Earth’s atmosphere, sustaining life and enabling various chemical reactions.
- Nitrogen (N<sub>2</sub>): Nitrogen gas constitutes the majority of Earth’s atmosphere, playing a crucial role in supporting life and maintaining atmospheric pressure.
- Hydrogen (H<sub>2</sub>): The lightest and most abundant element in the universe, hydrogen gas is prevalent in stars, planets, and interstellar space.
- Carbon Dioxide (CO<sub>2</sub>): Produced by respiration, combustion, and other natural processes, carbon dioxide gas plays a vital role in the Earth’s carbon cycle and greenhouse effect.
- Helium (He): A noble gas known for its inertness, helium is used in various applications, including cryogenics, helium balloons, and helium-neon lasers.
Special States of Matter
In addition to solids, liquids, and gases, there exist extraordinary states of matter with unique properties and behaviors. Among these, plasma stands out as a remarkable state, exhibiting fascinating characteristics that distinguish it from conventional forms of matter.
Plasma
Plasma is a state of matter characterized by ionized gas particles, where electrons are separated from atoms, resulting in a mixture of positively charged ions and negatively charged electrons. This ionization gives plasma distinct properties that set it apart from solids, liquids, and gases.
Properties of Plasma
Plasma possesses several unique properties, including:
- Electric Conductivity: Due to the presence of free-moving charged particles, plasma can conduct electricity, making it integral to various technological applications.
- Responsive to Electromagnetic Fields: Plasma interacts strongly with electromagnetic fields, allowing for manipulation and control in fusion reactors and other plasma-based devices.
- Luminosity: Many plasmas emit light, ranging from the glow of neon signs to the intense brilliance of stars, making plasma phenomena visible across the universe.
Examples of Plasma
Plasma is ubiquitous in the cosmos, manifesting in diverse forms and environments. Some notable examples include:
- Stars: The sun and other stars consist primarily of plasma, where nuclear fusion reactions occur, releasing vast amounts of energy and light.
- Auroras: Auroras, such as the Northern and Southern Lights, are natural light displays caused by the interaction of solar wind with the Earth’s magnetosphere, resulting in the ionization of gases in the atmosphere.
- Neon Signs: Neon signs and other gas-discharge lamps utilize plasma to produce colorful illumination, with electric currents exciting gas atoms to emit characteristic light.
- Fusion Reactors: Experimental fusion reactors harness the power of plasma to replicate the energy-producing processes of stars, offering the potential for clean and abundant energy generation.
Bose-Einstein Condensate (BEC)
Bose-Einstein Condensate (BEC) is a unique state of matter that emerges at ultra-low temperatures, near absolute zero. In this state, particles, known as bosons, occupy the same quantum state, forming a singular entity with remarkable properties.
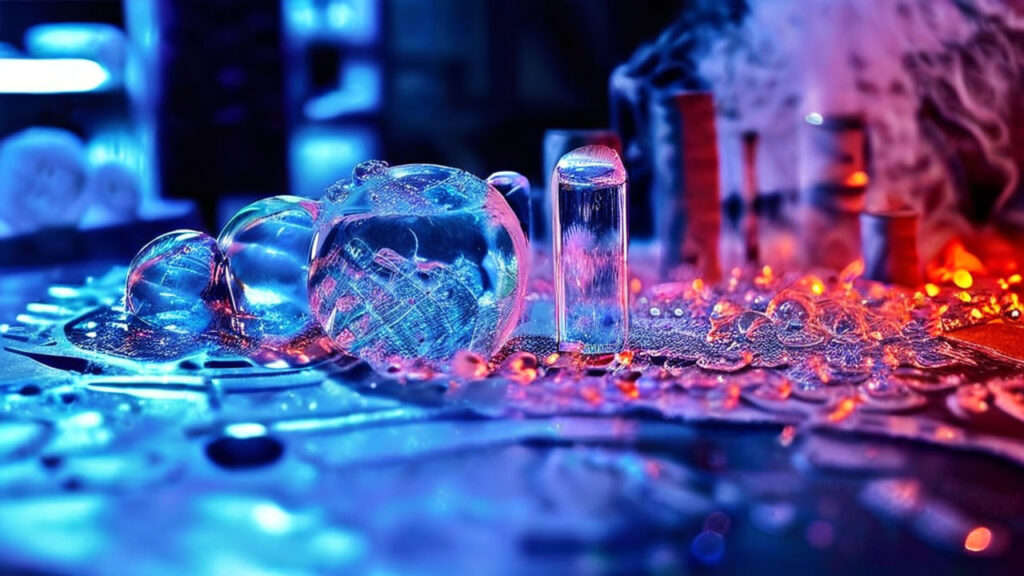
Properties of BEC
BEC exhibits several intriguing properties, including:
- Superfluidity: BEC displays superfluidity, meaning it flows without viscosity, exhibiting frictionless motion and the ability to pass through obstacles without losing energy.
- Quantum Coherence: Particles in BEC exhibit quantum coherence, behaving as a single quantum entity with wave-like characteristics, enabling phenomena such as interference and tunneling.
- Macroscopic Quantum Phenomena: Despite being composed of countless individual particles, BEC displays macroscopic quantum phenomena, allowing for the observation of quantum effects on a large scale.
Applications of BEC
BEC has found applications in various fields, including:
- Precision Measurement: BEC serves as a valuable tool in precision measurement devices, such as atomic clocks and interferometers, where its coherent properties enable unparalleled accuracy.
- Quantum Computing: The unique properties of BEC make it a promising candidate for quantum computing, where qubits encoded in the collective states of particles could revolutionize information processing.
- Fundamental Physics: BEC provides insights into fundamental physics phenomena, including the study of quantum mechanics and the behavior of matter at extremely low temperatures.
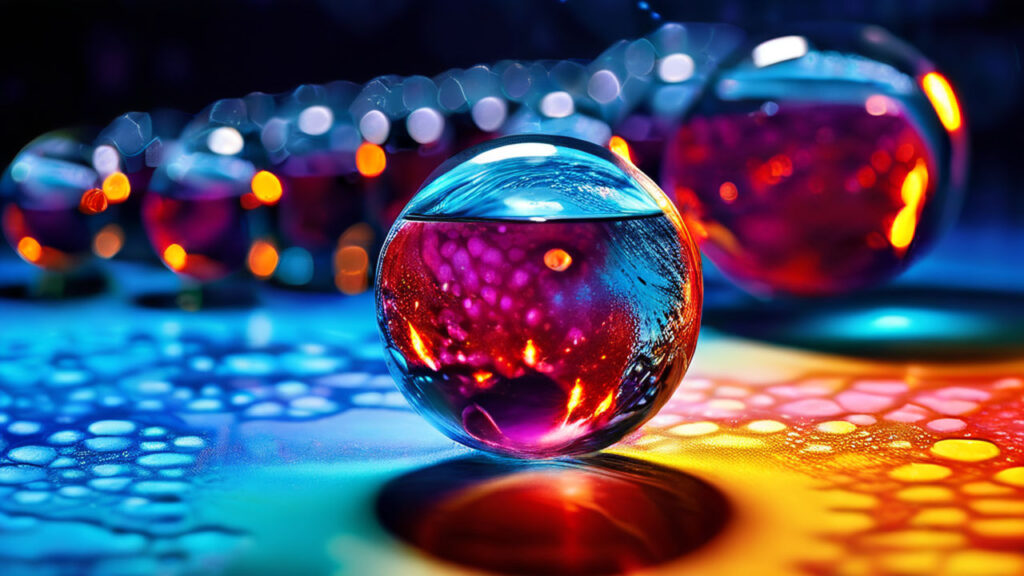
Fermionic Condensate
Fermionic Condensate is another exotic state of matter that arises at ultra-low temperatures, composed of fermions, particles with half-integer spin. Unlike BEC, which consists of bosons, fermionic condensates exhibit distinct properties and behaviors.
Properties of Fermionic Condensate
Fermionic Condensate possesses unique characteristics, such as:
- Fermionic Statistics: Fermions in condensates obey Fermi-Dirac statistics, leading to the Pauli exclusion principle, which prohibits multiple fermions from occupying the same quantum state.
- Quantum Degeneracy: At low temperatures, fermions in condensates occupy the lowest energy states, resulting in quantum degeneracy and collective behavior.
- Precision Measurement: Similar to BEC, fermionic condensates find applications in precision measurement devices, offering high sensitivity and stability.
Significance of Fermionic Condensate
The study of fermionic condensates holds significant implications for fundamental physics and technological applications:
- Quantum Technology: Fermionic condensates offer potential applications in quantum technology, including quantum computing, communication, and sensing, where the coherent behavior of fermions could enable novel functionalities.
- Fundamental Research: Fermionic condensates provide a platform for investigating fundamental physics phenomena, such as quantum entanglement, many-body physics, and the nature of quantum phase transitions.
Phase Transitions
Phase transitions refer to the changes in the physical state of matter that occur in response to variations in temperature, pressure, or other external factors. These transitions involve the rearrangement of particles and alterations in their interactions, leading to transformations between different states of matter.
Solid to Liquid (Melting)
The transition from a solid to a liquid, known as melting or fusion, occurs when the temperature of a substance rises above its melting point. During this process, the solid structure breaks down as the thermal energy provided by heat causes the particles to overcome their intermolecular forces, resulting in increased molecular mobility and the formation of a liquid.
Liquid to Gas (Vaporization)
Vaporization, also referred to as evaporation or boiling, is the phase transition from a liquid to a gas. This occurs when the temperature of a liquid reaches its boiling point, or when sufficient energy is supplied to the liquid to overcome the intermolecular forces holding it together. As the liquid molecules gain energy, they break free from the surface and enter the gas phase, forming vapor.
Solid to Gas (Sublimation)
Sublimation is the direct transition of a substance from the solid phase to the gas phase without passing through the intermediate liquid phase. This phenomenon occurs when the temperature and pressure conditions allow the solid particles to gain enough energy to overcome the intermolecular forces holding them together. As a result, the solid substance transforms directly into a gaseous state, bypassing the liquid phase entirely.
Theoretical States of Matter
In addition to the familiar states of matter observed in nature, theoretical physics predicts the existence of exotic states of matter that push the boundaries of our understanding of the universe. Among these theoretical states are quark-gluon plasma and strange matter, each offering tantalizing insights into the fundamental nature of matter.
Quark-Gluon Plasma
Quark-gluon plasma (QGP) is a hypothetical state of matter believed to have existed in the early universe, fractions of a second after the Big Bang, and under extreme conditions such as those created in high-energy particle collisions. In QGP, quarks and gluons, which are the fundamental building blocks of protons, neutrons, and other hadrons, are no longer confined within individual particles but instead exist freely within a hot and dense medium.
Strange Matter
Strange matter is another theoretical form of matter hypothesized to exist in the universe. It is composed of strange quarks, which are heavier and less stable than the up and down quarks that make up ordinary matter. According to the “strange matter hypothesis,” strange matter may be more stable than ordinary matter under certain conditions, potentially leading to the conversion of ordinary matter into strange matter in catastrophic events known as “strangelet conversion.”
Conclusion
In conclusion, the study of matter encompasses a vast and intricate tapestry of states, each with its own unique properties and behaviors. From the solidity of a rock to the ephemeral nature of plasma, matter manifests in diverse forms that shape the fabric of our universe. By delving into the fundamental states of matter, such as solids, liquids, and gases, as well as exploring exotic states like plasma and theoretical concepts such as quark-gluon plasma and strange matter, we gain a deeper understanding of the underlying principles that govern the cosmos.
As we continue to unravel the mysteries of matter through scientific inquiry and technological advancements, we unlock new frontiers of knowledge and discovery. Whether probing the depths of particle physics or harnessing the potential of quantum phenomena, the exploration of matter holds boundless possibilities for innovation and understanding.
In our quest to comprehend the nature of matter, let us remain curious, humble, and open to the wonders that lie ahead. For in the journey of discovery, we illuminate not only the mysteries of the universe but also the boundless potential of the human spirit.
Unique FAQs About the Different States of Matter
- What causes matter to change states?
- Matter changes states primarily due to variations in temperature and pressure. When heat is added or removed, or when pressure is altered, the intermolecular forces between particles can be overcome or strengthened, leading to transitions between solid, liquid, and gas states.
- Can matter exist in a state other than solid, liquid, or gas?
- Yes, matter can exist in other states beyond solids, liquids, and gases. Special states such as plasma, Bose-Einstein condensate (BEC), and fermionic condensate are examples of exotic forms of matter that exhibit unique properties and behaviors under specific conditions.
- What is the significance of studying different states of matter?
- Studying different states of matter is crucial for understanding the fundamental properties of materials and their behavior under various conditions. It provides insights into phenomena such as phase transitions, thermodynamics, and quantum mechanics, with applications ranging from materials science and engineering to cosmology and particle physics.
- Are there practical applications of understanding plasma in everyday life?
- Yes, understanding plasma has numerous practical applications in everyday life. Plasma technology is used in industries such as semiconductor manufacturing, sterilization, lighting (e.g., fluorescent and neon lights), and medicine (e.g., plasma-based surgical tools and plasma TVs).
- How do scientists create and study exotic states of matter in laboratories?
- Scientists create and study exotic states of matter in laboratories using specialized equipment and techniques. For example, they use particle accelerators and colliders to generate high-energy collisions, producing conditions similar to those present in the early universe to study quark-gluon plasma. Additionally, they employ ultra-low temperature environments and sophisticated cooling methods to create Bose-Einstein condensates and fermionic condensates for investigation.