- Introduction: Understanding the Basics of Protein Synthesis
- The Key Players in Protein Synthesis
- Transcription: From DNA to mRNA
- Translation: Decoding mRNA into Proteins
- Post-Translational Modifications
- Regulation of Protein Synthesis
- The Importance of Protein Synthesis in Health and Disease
- Current Research and Future Directions
- Conclusion: Piecing Together the Puzzle of Protein Synthesis
- FAQs (Frequently Asked Questions)
Introduction: Understanding the Basics of Protein Synthesis
Protein synthesis is a fundamental process within living organisms that involves the creation of proteins, vital molecules essential for various biological functions. Understanding the intricacies of protein synthesis is crucial for comprehending how life functions at the molecular level.
What is Protein Synthesis?
Protein synthesis refers to the biological process by which cells generate proteins from amino acids, following the instructions encoded in the DNA. This intricate process involves multiple steps and molecular components working together in a coordinated manner.
The Significance of Protein Synthesis in Living Organisms
Proteins play a plethora of essential roles in living organisms. They serve as structural components, enzymes facilitating biochemical reactions, hormones regulating physiological processes, and much more. Without protein synthesis, organisms would be unable to grow, repair damaged tissues, maintain cellular functions, or respond to their environment effectively.
Understanding the significance of protein synthesis sheds light on the fundamental mechanisms driving life itself. From the development of complex organisms to the functioning of individual cells, protein synthesis is indispensable for the survival and prosperity of all living beings.
The Key Players in Protein Synthesis
Protein synthesis is orchestrated by a trio of essential molecular players, each with its unique role in the process.
DNA: The Blueprint of Life
DNA, or deoxyribonucleic acid, serves as the blueprint of life, containing the genetic information necessary for the synthesis of proteins. The sequence of nucleotide bases in DNA determines the sequence of amino acids in proteins, making DNA the ultimate repository of genetic instructions. During protein synthesis, specific segments of DNA are transcribed into messenger RNA (mRNA), which carries the genetic code from the nucleus to the cytoplasm where protein synthesis occurs.
RNA: The Messenger of Genetic Information
RNA, or ribonucleic acid, acts as the intermediary messenger in protein synthesis. Different types of RNA molecules participate in various stages of protein synthesis, with messenger RNA (mRNA) playing a central role. mRNA carries the genetic information transcribed from DNA to the ribosomes, where it serves as a template for protein synthesis. Other types of RNA, such as transfer RNA (tRNA) and ribosomal RNA (rRNA), assist in decoding the mRNA sequence and catalyzing the formation of peptide bonds between amino acids, respectively.
Ribosomes: The Protein Factories
Ribosomes are complex molecular machines responsible for the actual synthesis of proteins. Composed of rRNA and protein subunits, ribosomes serve as the assembly line where amino acids are brought together and linked to form polypeptide chains. Ribosomes read the genetic code carried by mRNA and translate it into the corresponding sequence of amino acids, ultimately producing functional proteins with diverse roles in cellular processes.
Together, DNA, RNA, and ribosomes orchestrate the intricate dance of protein synthesis, ensuring the precise execution of genetic instructions and the production of proteins essential for life.
Transcription: From DNA to mRNA
Transcription is the first step in protein synthesis, where the genetic information encoded in DNA is transcribed into mRNA, which serves as a template for protein production.
Initiation: Unraveling the DNA
Transcription initiation begins with the unwinding of the DNA double helix at the target gene site. Enzymes called transcription factors recognize specific DNA sequences known as promoters, marking the starting point for transcription. RNA polymerase, the enzyme responsible for synthesizing RNA, binds to the promoter region, initiating the assembly of the transcription complex.
Elongation: RNA Polymerase at Work
Once the transcription complex is assembled, RNA polymerase moves along the DNA template, synthesizing mRNA in a process known as elongation. As RNA polymerase progresses, it unwinds the DNA double helix ahead of it and synthesizes an RNA molecule complementary to the DNA template. The growing mRNA strand is extended by adding nucleotides in a sequence dictated by the DNA template. As RNA polymerase advances, the DNA helix re-forms behind it, maintaining the integrity of the genetic code.
Termination: The Completion of mRNA Synthesis
Transcription terminates when RNA polymerase reaches a specific DNA sequence known as the terminator. The terminator sequence signals the end of the gene and triggers the release of the newly synthesized mRNA molecule. As RNA polymerase dissociates from the DNA template, the transcription complex disassembles, and the mRNA transcript is released into the nucleus. The completed mRNA molecule undergoes further processing, such as capping, splicing, and polyadenylation, before it is ready for export to the cytoplasm for translation into proteins.
Transcription is a tightly regulated process that ensures the accurate transcription of genetic information from DNA to mRNA, laying the foundation for protein synthesis and cellular function.
Translation: Decoding mRNA into Proteins
Translation is the process by which the genetic information encoded in mRNA is deciphered and used to assemble proteins.
Initiation: The Start Codon
Translation initiation begins with the recognition of a specific sequence of nucleotides on the mRNA molecule known as the start codon. In most cases, the start codon is AUG, which codes for the amino acid methionine. The small ribosomal subunit binds to the mRNA molecule at the start codon, positioning the ribosome for protein synthesis initiation. Initiation factors assist in the recruitment of the large ribosomal subunit, forming a functional ribosome ready to begin translation.
Elongation: The Role of tRNA and Ribosomes
During translation elongation, amino acids are added to the growing polypeptide chain according to the mRNA sequence. This process requires the coordinated action of transfer RNA (tRNA) molecules and ribosomes. Each tRNA molecule carries a specific amino acid and contains an anticodon region that recognizes and base-pairs with the corresponding codon on the mRNA. As the ribosome moves along the mRNA template, it catalyzes the formation of peptide bonds between adjacent amino acids, linking them together to form a polypeptide chain. The ribosome advances along the mRNA molecule, synthesizing the polypeptide in a sequential manner until it reaches a stop codon.
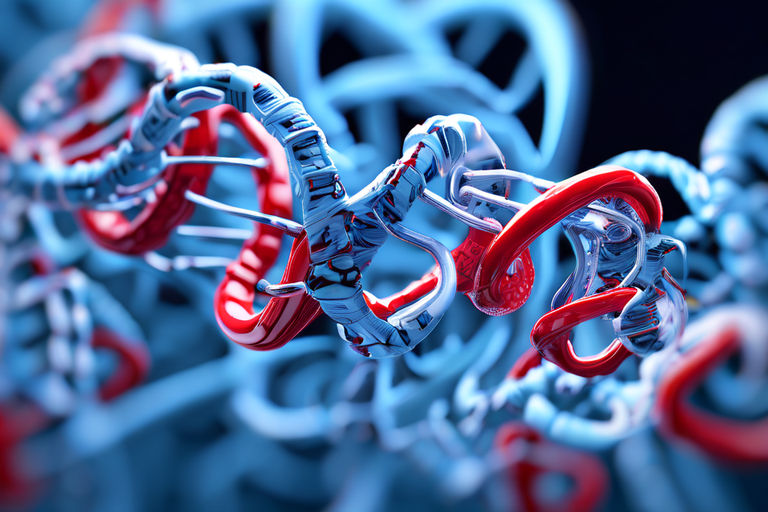
Termination: Reaching the Stop Codon
Translation termination occurs when the ribosome encounters a stop codon (UAA, UAG, or UGA) on the mRNA molecule. Stop codons do not code for any amino acids but instead signal the termination of protein synthesis. When a stop codon is reached, release factors bind to the ribosome, causing the release of the completed polypeptide chain from the ribosome. The ribosome dissociates from the mRNA molecule, and the newly synthesized protein is released into the cytoplasm, where it undergoes further folding and processing to attain its functional conformation.
Translation is a highly orchestrated process that requires the precise coordination of mRNA, tRNA, ribosomes, and various translation factors. By decoding the genetic information contained within mRNA, translation enables the synthesis of proteins essential for cellular function and organismal survival.
Post-Translational Modifications
Post-translational modifications (PTMs) are chemical modifications that occur to proteins after they have been synthesized. These modifications play crucial roles in regulating protein structure, function, and localization within the cell.
Folding and Processing of Proteins
After synthesis, many proteins undergo folding into their functional three-dimensional structures. Protein folding is a complex process guided by chaperone proteins, which assist in proper folding and prevent misfolding or aggregation. Additionally, proteins may undergo processing steps, such as cleavage of signal peptides or removal of amino acid residues, to achieve their mature form. Proper protein folding and processing are essential for ensuring protein functionality and preventing the accumulation of misfolded proteins, which can lead to cellular dysfunction and disease.
Adding Functional Groups
Proteins can undergo various PTMs involving the addition of chemical groups to specific amino acid residues. Common modifications include phosphorylation, glycosylation, acetylation, methylation, and ubiquitination, among others. These modifications can alter protein activity, stability, localization, and interactions with other molecules. For example, phosphorylation of serine, threonine, or tyrosine residues can regulate enzyme activity or signal transduction pathways, while glycosylation can modulate protein stability or cell-cell recognition processes. PTMs add a layer of complexity to protein regulation and contribute to the diversity of protein functions within cells.
Targeting Proteins to Specific Cellular Locations
Many proteins must be targeted to specific cellular compartments or organelles to perform their functions effectively. This targeting is facilitated by signal sequences or localization signals present within the protein sequence. For example, proteins destined for secretion or insertion into the plasma membrane contain signal peptides that direct them to the endoplasmic reticulum (ER) for processing and trafficking. Similarly, proteins destined for the nucleus or mitochondria contain nuclear localization signals (NLS) or mitochondrial targeting sequences (MTS), respectively, which guide their import into these organelles. Proper targeting ensures that proteins are localized to their functional sites within the cell, allowing for efficient execution of cellular processes.
Post-translational modifications play diverse and critical roles in regulating protein structure, function, and localization within cells. By modulating protein activity, stability, and localization, PTMs contribute to the complexity and versatility of cellular processes and play key roles in health and disease.
Regulation of Protein Synthesis
Protein synthesis is tightly regulated by a variety of mechanisms to ensure that the right proteins are produced in the right amounts at the right times. Regulation occurs at multiple levels, including transcription, translation, and post-translational modification, and is influenced by both intrinsic and extrinsic factors.
Controlling Transcription and Translation
One of the primary means of regulating protein synthesis is through the control of transcription and translation. Transcriptional regulation involves the modulation of RNA polymerase activity and the accessibility of DNA to transcription factors, which can either promote or inhibit gene expression. Similarly, translational regulation involves the modulation of ribosome function and the availability of mRNA and tRNA molecules, which can affect the rate at which proteins are synthesized. These regulatory mechanisms allow cells to respond dynamically to changing environmental conditions and developmental cues by adjusting the expression of specific genes.
Feedback Mechanisms
Feedback mechanisms play a critical role in fine-tuning protein synthesis to maintain cellular homeostasis. Negative feedback loops, in which the end product of a biochemical pathway inhibits its own production, are common regulatory mechanisms in protein synthesis. For example, certain proteins may act as repressors or inhibitors of transcription or translation, shutting down protein synthesis when sufficient levels of the protein have been produced. Positive feedback loops, in which the end product of a pathway stimulates its own production, can also play a role in amplifying cellular responses to external stimuli.
Impact of Environmental Factors
Environmental factors such as temperature, pH, nutrient availability, and stress can profoundly impact protein synthesis. Cells must adapt their protein synthesis machinery in response to changes in environmental conditions to maintain cellular function and survival. For example, heat shock proteins are synthesized in response to elevated temperatures to help protect cells from protein denaturation and damage. Similarly, nutrient availability can influence the expression of genes involved in nutrient metabolism and uptake, allowing cells to optimize protein synthesis according to their metabolic needs.
Overall, the regulation of protein synthesis is a complex and dynamic process that allows cells to respond to internal and external cues and maintain cellular homeostasis. By controlling transcription and translation, employing feedback mechanisms, and responding to environmental factors, cells can finely tune protein synthesis to meet their metabolic demands and adapt to changing conditions.
The Importance of Protein Synthesis in Health and Disease
Protein synthesis is essential for the maintenance of health and plays a critical role in the development, function, and regulation of biological systems. Dysregulation of protein synthesis can lead to various disease states, highlighting the importance of understanding and targeting this fundamental process in therapeutics.
Protein Synthesis and Growth
Protein synthesis is intimately linked with growth and development in organisms of all sizes, from single-celled organisms to multicellular organisms like humans. During periods of growth, such as embryonic development, childhood, and adolescence, protein synthesis rates are typically elevated to support tissue growth, organ development, and overall body growth. Proteins serve as structural components in cells, tissues, and organs, providing the framework necessary for growth and repair. Additionally, proteins play key roles in signaling pathways that regulate growth and development, ensuring that these processes occur in a coordinated and controlled manner.
Protein Synthesis in Disease States
Dysregulation of protein synthesis is implicated in numerous disease states, ranging from genetic disorders to cancer and neurodegenerative diseases. Mutations or abnormalities in genes encoding proteins involved in the protein synthesis machinery can disrupt normal cellular processes and lead to disease. For example, mutations in genes encoding ribosomal proteins or translation factors can impair ribosome function and protein synthesis, resulting in developmental disorders known as ribosomopathies. In cancer, dysregulated protein synthesis is a hallmark feature, as cancer cells often exhibit increased protein synthesis rates to support their rapid proliferation and growth. Moreover, aberrant protein synthesis is observed in neurodegenerative diseases such as Alzheimer’s disease and Parkinson’s disease, where misfolded proteins accumulate and disrupt cellular function.
Targeting Protein Synthesis in Therapeutics
Given the critical role of protein synthesis in health and disease, targeting this process has emerged as a promising strategy for therapeutic intervention. Therapies aimed at modulating protein synthesis can be used to treat a wide range of conditions, including cancer, infectious diseases, and genetic disorders. For example, inhibitors of specific steps in protein synthesis, such as antibiotics that target bacterial ribosomes or drugs that inhibit translation initiation factors in cancer cells, can selectively inhibit the growth and proliferation of diseased cells while sparing healthy cells. Additionally, gene therapy approaches aimed at correcting mutations in genes encoding proteins involved in protein synthesis hold promise for treating genetic disorders caused by defects in the protein synthesis machinery.
In summary, protein synthesis is a fundamental process that underlies health and disease. Understanding the intricacies of protein synthesis and its role in cellular function and disease pathology is crucial for the development of novel therapeutic strategies aimed at restoring normal protein synthesis and improving patient outcomes.
Current Research and Future Directions
Research into protein synthesis continues to advance our understanding of this fundamental biological process and its implications for health, disease, and biotechnology. Exciting developments in technology and methodology are driving progress in the field, opening up new avenues for exploration and application.
Advancements in Understanding Protein Synthesis
Recent advancements in molecular biology, structural biology, and bioinformatics have provided unprecedented insights into the mechanisms and regulation of protein synthesis. High-resolution imaging techniques, such as cryo-electron microscopy (cryo-EM), have allowed researchers to visualize ribosomes and other components of the protein synthesis machinery at near-atomic resolution, revealing the intricate molecular mechanisms underlying translation. Additionally, advances in sequencing technologies and computational modeling have facilitated the study of mRNA dynamics, RNA-protein interactions, and the regulation of translation at the genome-wide level. These advancements are deepening our understanding of how protein synthesis is regulated in health and disease and providing new targets for therapeutic intervention.
Emerging Technologies in Studying Protein Synthesis
Emerging technologies are revolutionizing the study of protein synthesis by enabling researchers to probe translation dynamics with unprecedented precision and sensitivity. For example, single-molecule imaging techniques, such as single-molecule fluorescence microscopy and nanopore sequencing, allow researchers to monitor translation events in real-time at the level of individual molecules. These approaches provide insights into the kinetics of translation, the role of ribosome heterogeneity, and the impact of regulatory factors on translation efficiency. Moreover, advances in genome editing technologies, such as CRISPR-Cas9, are enabling researchers to manipulate the expression of specific genes involved in protein synthesis with unprecedented precision, facilitating the study of gene function and regulation in diverse biological contexts.
Implications for Medicine and Biotechnology
The insights gained from research on protein synthesis have far-reaching implications for medicine and biotechnology. Understanding the molecular mechanisms underlying protein synthesis is essential for developing novel therapeutics targeting translation in cancer, infectious diseases, and genetic disorders. For example, drugs that selectively inhibit translation in cancer cells while sparing healthy cells are being explored as potential anticancer agents. Moreover, advancements in biotechnology are enabling the production of recombinant proteins for therapeutic use, biopharmaceutical production, and industrial applications. By harnessing the power of protein synthesis, researchers are unlocking new possibilities for the diagnosis, treatment, and prevention of disease, as well as the development of innovative biotechnological solutions to address global challenges in healthcare, agriculture, and environmental sustainability.
In summary, current research on protein synthesis is paving the way for exciting discoveries and innovations with profound implications for medicine, biotechnology, and our understanding of life itself. By leveraging cutting-edge technologies and interdisciplinary approaches, researchers are unraveling the mysteries of protein synthesis and harnessing its potential to address some of the most pressing challenges facing society today.
Conclusion: Piecing Together the Puzzle of Protein Synthesis
Protein synthesis stands as a cornerstone of life, orchestrating the intricate molecular ballet within cells and influencing the broader realms of growth and health. Delving into the complexities of protein synthesis unveils the inner workings of biology, offering glimpses into the fundamental mechanisms that drive cellular function and organismal development.
Through the study of protein synthesis, researchers gain valuable insights into disease mechanisms, paving the way for the development of innovative therapeutics and diagnostic tools. By deciphering the molecular intricacies of translation and transcription, scientists can identify novel targets for intervention and design precision medicines tailored to specific disease states.
Moreover, the exploration of protein synthesis pushes the boundaries of scientific knowledge, driving advancements in technology and methodology. From high-resolution imaging techniques to single-molecule manipulation tools, researchers continue to refine their understanding of protein synthesis and its implications for health, disease, and biotechnology.
In essence, protein synthesis serves as a linchpin connecting the molecular foundations of life with the broader landscape of biological complexity. By unraveling its mysteries and piecing together its puzzle, we not only deepen our understanding of biology but also unlock new possibilities for improving human health and advancing scientific discovery.
FAQs (Frequently Asked Questions)
- What is the primary role of DNA in protein synthesis?
- DNA serves as the blueprint for protein synthesis, containing the genetic information needed to produce specific proteins.
- How does transcription differ from translation?
- Transcription involves the synthesis of mRNA from DNA, while translation is the process of converting mRNA into proteins.
- What are some examples of post-translational modifications?
- Post-translational modifications include adding functional groups, cleaving proteins, and targeting proteins to specific cellular locations.
- Why is protein synthesis regulation important?
- Regulation of protein synthesis ensures that cells produce the right proteins in the right amounts at the right time, contributing to cellular homeostasis and function.
- How does protein synthesis relate to human health?
- Protein synthesis is essential for growth, development, and maintaining cellular functions. Dysregulation of protein synthesis can lead to various diseases, including cancer and neurodegenerative disorders.